Липосомальная наномедицина:приложения для доставки лекарств в терапии рака
Аннотация
Растущая распространенность рака, болезни, при которой быстрый и неконтролируемый рост клеток вызывает осложнения и дисфункцию тканей, является одной из серьезных и напряженных проблем ученых и врачей. В настоящее время диагностика рака и особенно его эффективное лечение считаются одной из самых серьезных проблем в области здравоохранения и медицины в прошлом веке. Несмотря на значительные успехи в открытии и доставке лекарств, их многочисленные побочные эффекты и недостаточная специфичность и чувствительность, которые обычно вызывают повреждение здоровых тканей и органов, являются серьезными препятствиями при их использовании. Ограничение продолжительности и количества введения этих терапевтических агентов также является проблемой. С другой стороны, распространение опухолевых клеток, устойчивых к типичным методам лечения рака, таким как химиотерапия и лучевая терапия, подчеркивает острую потребность в инновациях, улучшении и развитии свойств противоопухолевых препаратов. Липосомы были предложены в качестве подходящего кандидата для доставки лекарств и лечения рака в наномедицине из-за их способности хранить лекарства с различными физическими и химическими характеристиками. Более того, высокая гибкость и потенциал структуры липосом для химической модификации за счет конъюгирования различных полимеров, лигандов и молекул является важным преимуществом липосом не только для усиления их фармакологических свойств, но и для повышения эффективности противоопухолевых препаратов. Липосомы могут повысить чувствительность, специфичность и стойкость этих противораковых клеточных агентов в организме и обеспечить замечательные преимущества для применения в наномедицине. Мы рассмотрели открытие и разработку липосом, сосредоточив внимание на их клиническом применении для лечения различных видов рака и заболеваний. Также были рассмотрены и обсуждены способы улучшения свойств липосомальных препаратов, а также их возможности и проблемы для лечения рака.
Графический аннотация
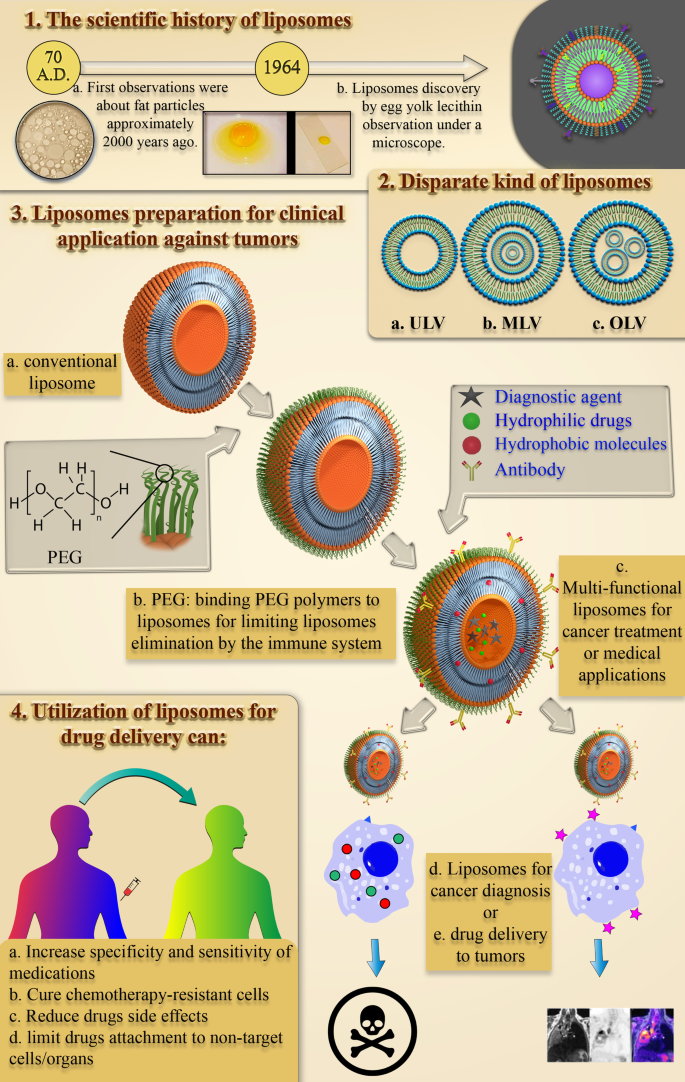
Введение
Рак, заболевание, при котором здоровые клетки организма выходят из нормального состояния и бесконтрольно делятся, признан серьезной медицинской проблемой в текущем веке. Это осложнение вызвано накоплением канцерогенов в окружающей среде или генетическими мутациями [1] и признано серьезной медицинской проблемой в текущем столетии. Ежегодно миллионы людей умирают от рака, а количество новых пациентов и уровень смертности постоянно растут [2]. Согласно отчетам Всемирной организации здравоохранения (ВОЗ), рак был второй ведущей причиной смертности в 2018 году, и оценка показала, что в том году от различных видов рака умерло около 9,6 миллиона человек. В 2018 году каждая шестая смерть была примерно вызвана раком. Около 70% смертей от рака происходит в развивающихся странах и странах с низким уровнем доходов. Однако следует также учитывать заболеваемость и смертность от рака в развитых странах [3].
Известно, что химиотерапия противоопухолевыми средствами является важным методом лечения рака [4]. Химиотерапия бесплатными лекарствами ограничена из-за отсутствия соответствующей чувствительности и специфичности. В результате это ограничение препятствовало точному лечению из-за побочных эффектов и подавляло проявление достаточного противоопухолевого эффекта [5]. Химиоиммунотерапия, сопутствующее комбинированное лечение, также была предложена в качестве эффективного и многообещающего метода лечения рака, направленного на лечение опухолевых клеток, устойчивых к обычным лекарствам. В последние годы для лечения рака были открыты и применены различные традиционные и современные методы лечения. Например, для уменьшения побочных эффектов обычных противоопухолевых препаратов, в частности химиотерапевтических агентов, различных наномедицинских препаратов [6], включая вирусные наночастицы (ВНЧ) [7, 8], квантовые точки [9], полимерные наноматериалы [10] и липосомы. [11] были применены.
Среди различных наномедицинских препаратов липосомы в виде сферических наночастиц (НЧ) имеют особую структуру. Присутствие двух водных и органических фаз в липосомном компоненте позволяет захватывать оба вида гидрофильных и гидрофобных агентов и создает заметное преимущество липосом перед многими наноносителями. Один из способов повысить специфичность, биодоступность и биосовместимость противоопухолевых препаратов - заключить их в липосомы различных типов [5]. За последние два десятилетия были предприняты значительные усилия по использованию липосом в терапевтических целях. Некоторые из этих препаратов, такие как DaunoXome® и Caelyx®, были одобрены для общего и клинического применения, тогда как другие находятся на окончательной стадии производства и утверждения [12].
Как правило, существуют различные виды терапевтических липосом, такие как иммунолипосомы и pH-чувствительные липосомы. Иммунолипосомы представляют собой большую группу наномедицинских устройств, также известных как системы адресной доставки лекарств (DDS), которые продемонстрировали значительные противораковые эффекты в исследованиях и исследованиях [13]. pH-чувствительные липосомы также известны как группа полиморфных липосом, в которых структура и составляющие молекулы изменяются при изменении pH, что приводит к высвобождению содержащихся в них лекарств [14]. Более того, липосомы, как и другие наномедицины, могут быть использованы для восстановления и регенерации тканей, визуализации и диагностики в дополнение к системам доставки лекарств. Использование липосом в различных аспектах упрощает идентификацию, ведение и лечение заболеваний и рака [15].
В этой статье приводится краткое изложение результатов открытия и структуры липосом, различных свойств липосом и липосомальных лекарств для лечения рака, представленных на рынке, и их разработки. В конечном итоге будет завершен отчет о возможностях и проблемах использования липосомальных наномедицинских препаратов, который можно выделить как важнейшие вопросы, которые следует отметить в будущих исследованиях ученых, что приведет к устранению ограничений и усилению положительных моментов.
Основной текст
Научная история липосом:открытие и определение
Прошло около 1950 лет от ранних исследований структуры и поведения маленьких липидных частиц в водной среде до первой одобренной FDA США наночастицы для доставки лекарств на липидной основе. Процесс изучения поведения липидов и жировых частиц в воде начался с первых наблюдений Плиния Старшего почти 2000 лет назад [16]. В конце семнадцатого века открытие клетки Энтони Ван Хук подняло много вопросов о структуре клеток [17]. Затем Гортер и Грендель обнаружили присутствие фосфолипидных бислоев в клеточных мембранах [18]. Впоследствии Сингер и Николсон описали модель двухслойной мозаичной мембраны для объяснения поведения фосфолипидов клеточной мембраны [19]. Эти научные наблюдения и гипотезы привлекли внимание других ученых к полученным из жира NPs. В 1960-х Алек Д. Бэнгхэм, изучавший влияние липидов, особенно фосфолипидов, на процесс свертывания крови в Институте Бабрахама [20, 21], случайно наблюдал первые липосомы и был удивлен, увидев спонтанные сферические частицы, образующиеся в них. вода [22]. Позже Джеральд Вайсманн, посетитель лаборатории Алека Бэнгхэма, который был осведомлен о результатах исследования Бэнгхэма, назвал наблюдаемую Алеком смектическую мезофазу «липосомами», а не «бангосомами», и был удостоен Нобелевской премии [22]. Научная история открытия липосом представлена на рис. 1.
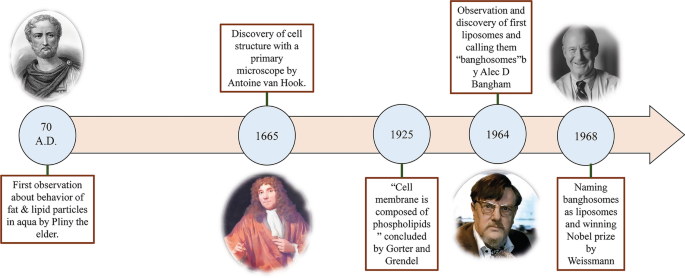
Схема наблюдений, которые привели к открытию липосом. Исторические и научные тенденции изучения поведения липидных и жировых частиц в воде и наблюдения, приведшие к открытию липосом, а также изображения ученых, участвовавших в этом событии, Плиния Старшего [23], Энтони Ван Хука [24], Алека Д. Бэнгхэм [25] и Джеральд Вайсманн [26], соответственно, слева направо
Какие структуры сегодня известны как липосомальные наночастицы?
Сейчас предпринимаются активные усилия по определению липосомных НЧ и разумному открытию их свойств. В настоящее время липосомы определяются как спонтанно образующиеся сферические фрагменты, которые состоят из двухслойной липидной мембраны и гидрофильного ядра.
Липосомы различаются по размеру от 10 до 2500 нм (или 2,5 мкм) [15]. Однако большинство липосом, вводимых для доставки лекарств, обычно имеют размер примерно от 50 до 450 нм. Безусловно, липосомы с гораздо большими размерами также могут быть использованы в медицинских целях [27]. Кроме того, липосомы в основном состоят из фосфолипидов. Фосфолипиды - это липиды, которые очень похожи на триглицериды. В структуре фосфолипидов есть гидрофильный полюс и две гидрофобные цепи. Таким образом, фосфолипиды считаются амфифильными молекулами.
Липосомная мембрана фосфолипидов в основном включает фосфатидилхолин (PC), сфингомиелин (SM), фосфатидилсерин (PS) и фосфатидилэтаноламин (PE), которые являются амфифильными и имеют сильную тенденцию к образованию определенных структур в воде [28]. Физическая причина этого явления - совместное присутствие гидрофильной головки (молекула фосфата) и двух гидрофобных хвостов (жирных кислот) в фосфолипидах. Фосфатная группа взаимодействует с H 2 O полярные молекулы, а гидрофобные хвосты ускользают от молекул воды и взаимодействуют друг с другом [29]. В этом случае неполярные цепи располагаются друг напротив друга и образуют бислой, создавая между ними липофильное пространство. Соответственно, эта липофильная часть структуры липосом может применяться для хранения гидрофобных агентов и материалов. Более того, гидрофильная часть фосфолипида затем направляется к молекулам воды посредством молекулярных сил, таких как водородные связи, Ван-дер-Ваальс и т. Д., Которые возникают между ними. Это приводит к образованию гидрофильной области внутри липосом. Структура молекулы лецитина как природного фосфолипида, который содержится в большом количестве в яичном желтке и может образовывать липосомы в воде и различных участках липосомы, показана на рис. 2.
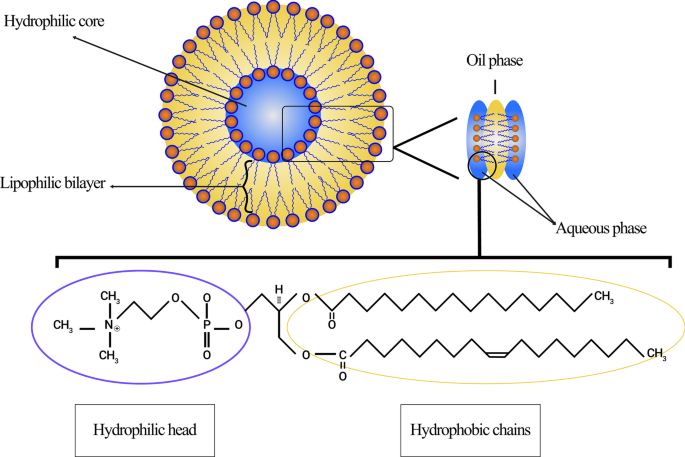
Схематическое изображение липосом, происходящих из лецитина. Показаны различные области липосом, включая гидрофильное ядро и гидрофобный бислой. Уточняется структура молекулы лецитина, ее гидрофильный полюс и гидрофобные цепи
Кроме того, сферическая структура липосом после растворения в воде в зависимости от типа молекул, температуры водной среды, молярной концентрации и присутствия других веществ, таких как ионы, определяет ее окончательную форму [30]. Преобладающие физические и химические свойства липосомы - это чистые свойства входящих в нее липидов, особенно фосфолипидов и других молекул, составляющих ее. Эти свойства включают проницаемость, плотность поверхностного заряда и общий размер [31].
Несопоставимые типы классификации липосом
С момента открытия липосом эти структуры всегда использовались как важная часть биологических, биофизических, биохимических или фармацевтических исследований.
Сегодня липосомы можно разделить на категории в зависимости от их размера, количества фосфолипидных бислоев, процедуры синтеза и механизма получения. По размеру липосомы можно разделить на три группы:маленькие, средние и большие. Учитывая количество мембранных слоев, они могут быть однослойными везикулами (ULV), олиголамеллярными везикулами (OLV) и многослойными везикулами (MLV). В этом отношении ULV представляют собой липосомы, состоящие из одного фосфолипидного бислоя размером примерно от 50 до 250 нм, тогда как MLV намного больше, примерно 0,5–1,5 мкм, и включают несколько фосфолипидных бислойных мембран [32]. Различные методы синтеза приводят к разнице между этими двумя группами. С точки зрения применения, УМО также имеют большую внутреннюю гидрофильную среду, что делает их пригодными для улавливания гидрофильных лекарственных средств. Небольшие однослойные везикулы (SUV), как и ULV, состоят из одного фосфолипидного бислоя, но по размеру они меньше 100 нм [33, 34]. С морфологической точки зрения OLV представляют собой липосомы, которые состоят из двух-пяти везикул, которые могут иметь одинаковые или разные размеры. В структуре OLV все везикулы заключены в один большой фосфолипидный бислой, но не находятся внутри друг друга. OLV обычно составляют около 0,1–1 мкм [33,34,35]. В отличие от СМО, МЖВ не идеальны для доставки гидрофильных веществ. MLV в основном используются для доставки гидрофобных агентов [36]. Различные типы липосом показаны на рис. 3.
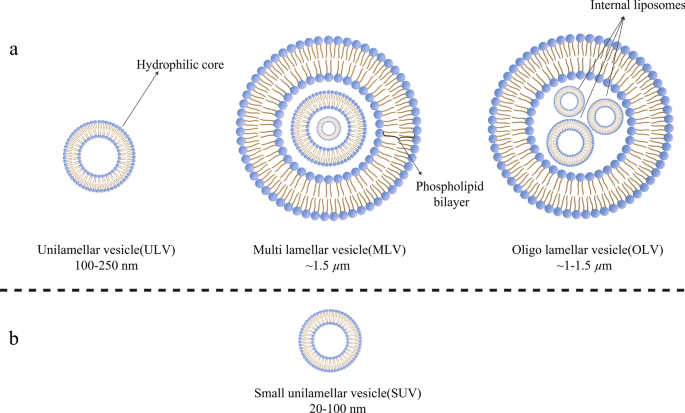
Классификация липосом по разным критериям: а Липосомы делятся на три категории по размеру; б Структура небольших однослойных пузырьков (SUV), входящих в состав однослойных пузырьков (ULV), которые имеют заметный небольшой размер
Методы приготовления липосом и разработка их новых поколений
В отличие от наночастиц золота в виде твердых НЧ липосомы являются мягкими НЧ [37] и могут быть синтезированы различными методами. Например, у MLV и ULV есть разные механизмы приготовления. В большинстве этих методов для растворения липидов, предназначенных для образования липосомных мембран (с желаемым молярным соотношением) в круглодонной колбе (RBF), используется конкретный растворитель (такой как хлороформ или метанол и т. Д.). Например, рукопожатие - это основная процедура для синтеза MLV [38]. Во время этой процедуры, которая также известна как гидратация липидной пленки, липиды добавляются к органическому растворителю. Затем растворитель выпаривают на роторном устройстве, а твердый продукт липолизируют. В конечном итоге липосомы синтезируются методами гидратации и экструзии [39]. Другие методы синтеза липосом включают обработку ультразвуком, испарение с обращенной фазой, ячейку французского давления, сублимационную сушку и мембранную экструзию [38, 40].
Кроме того, липосомы также можно упорядочить по различным категориям в зависимости от их открытия и развития с течением времени. Липосомы первого поколения обычно называют обычными или классическими липосомами. Проблемы, которые наблюдались при использовании обычных липосом в качестве терапевтических НЧ, были выявлены так быстро in vivo. Одним из первых изученных вопросов было ограничение захвата лекарства липосомами. Другими словами, многие лекарства не могли храниться в липосомах первого поколения [41]. Наряду с большим желанием изучить структуру и свойства липосом, такие как стабильность, терапевтическая эффективность и возможность клинического применения, эти проблемы привели к разработке липосом второго поколения за счет изменения составляющих липидов, поверхностного заряда, веса нетто и т.д. и общий объем [42]. Если быть точным, липосомы второго поколения в основном синтезируются путем добавления некоторых гидрофильных полимеров к обычным липосомам для увеличения срока их хранения в жидкостях организма, чтобы сделать их подходящими кандидатами для систем доставки лекарств. Этот вид липосом можно разделить на две группы:неспецифические липосомы с длительной циркуляцией или липосомы с длительной циркуляцией, нацеленные на лиганд [43].
Археосомы как новое поколение липосом состоят из липидов мембран архей и синтетических аналогов фосфолипидов. В последнее десятилетие были предприняты обширные и значительные усилия по исследованию потенциала археосом для использования в доставке лекарств и вакцин. Структурные ядра липидов архейного типа представляют собой молекулы диэфиров или тетраэфиров с насыщенными фитанильными цепями, которые содержат от 20 до 40 атомов углерода. Эти углеродные цепи присоединяются к эфирным связям sn-2,3-атомов углерода глицерина основной цепи, обнаруженного в археолах или кальдархеолах. Как упоминалось выше, эти частицы могут также широко использоваться для доставки лекарств при неопластических осложнениях, аллергиях и инфекциях, а также для вакцинации [44].
Оценка характеристик биоматериала и физико-химических свойств липосом
Как упоминалось ранее, несмотря на широкие достижения в области медицины, лечение некоторых заболеваний, особенно рака, по-прежнему сталкивается с серьезными проблемами из-за неэффективных терапевтических агентов и методов. Корректировка дозы вводимого лекарства для воздействия на опухоль - сложный вопрос из-за узкого терапевтического окна противораковых средств. Другими словами, небольшое расстояние между терапевтической и токсической дозами, а также несоответствующая чувствительность и специфичность создали большой спрос на передовые лечебные процедуры [42].
Более того, в последнее время внимание привлекло использование наноматериалов для доставки лекарств в ткани. Биосовместимость и биоразлагаемость - это две важные характеристики биоматериалов, используемые в системах доставки. Биосовместимость необходима для предотвращения повреждения терапевтическими НЧ тканей и систем организма, а биоразлагаемость необходима для расщепления НЧ на нетоксичные соединения и простого удаления их из органов [15]. После обнаружения липосом ученые начали применять их в качестве наноматериалов для доставки лекарств. Как уже упоминалось, липосомы обладают двумя необходимыми свойствами биоматериала для терапевтических целей:биосовместимостью и биоразлагаемостью [36]. Кроме того, липосомальные НЧ обладают другими характеристиками, которые делают их пригодными для этой цели. Например, из-за особой структуры липосом в них могут быть инкапсулированы как группы гидрофильных (водорастворимых), так и гидрофобных (липидорастворимых). Кроме того, наличие двухслойной фосфолипидной мембраны в липосомах защищает агенты, хранящиеся в липосомах, от различных явлений и повреждений, таких как деградация ферментов, биологическая инактивация иммунологическими структурами и химические изменения in vivo. У этого пункта есть два важных преимущества:во-первых, структура молекул, заключенных в липосоме, сохраняется до достижения целевой ткани, и в нее не вносятся никакие модификации, и, во-вторых, другие здоровые и нецелевые ткани защищены от воздействия лекарство из-за липосомальной мембраны и не может быть подвержено влиянию этих агентов [42]. Липосомы также можно применять для доставки генетических материалов, таких как ДНК, РНК и т.д., а также в целях генной терапии. Липосомы, используемые для этой цели, могут состоять из катионных, анионных, нейтральных липидов и фосфолипидов или их смеси [45]. Некоторые диагностические агенты и средства визуализации, такие как углеродные точки, можно использовать для обнаружения рака и визуализации в комбинации липосом или по отдельности [46]. Хотя углеродные точки частично одобрены для клинического применения и используются в исследованиях, цитотоксичность остается серьезным препятствием для их широкого применения [47]. Общая структура НЧ липосомальных лекарственных средств представлена на рис. 4.
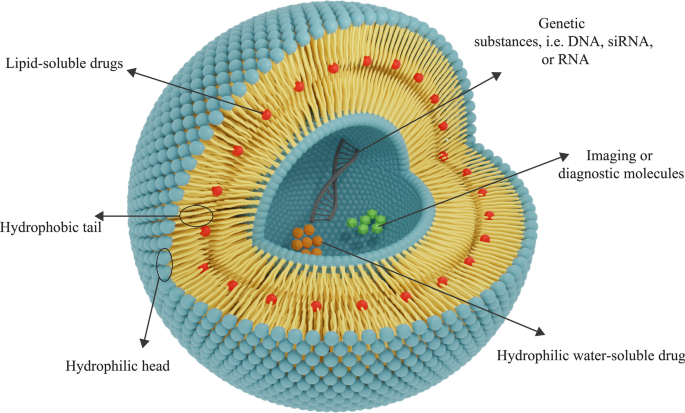
Общая структура липосом состоит из слоев фосфолипидов. В зависимости от гидрофильности-гидрофобности лекарственного средства будет определяться подходящий вид липосом для его доставки. Гидрофильные лекарства захватываются центральным гидрофильным ядром, а гидрофобные лекарства помещаются в липофильную область. Липосомы также можно использовать для доставки генов
Мембранообразующие фосфолипиды, присутствующие в липосомах, являются нетоксичными соединениями и могут быть синтезированы в широком диапазоне размеров. Физико-химические свойства липосом зависят от их составляющих. Таким образом, липосомы с желаемыми свойствами могут быть синтезированы путем добавления определенных соединений, таких как холестерин, полиэтиленгликоль (ПЭГ) и т. Д. Кроме того, мембрана липосом непроницаема для гигантских молекул, что помогает лучше удерживать материал внутри липосомы [48 ]. Все упомянутые особенности липосомы делают ее подходящим наноматериалом для использования в доставке терапевтических агентов для лечения различных заболеваний, особенно рака.
Грегори Грегориадис, как один из пионеров в этой области, предложил гипотезу использования липосом для систем доставки лекарств и заявил, что лекарственные соединения могут захватываться липосомами [49]. Сообщалось о соответствующих биоматериалах и физико-химических свойствах липосом. Например, исследование липосом, содержащих противоопухолевый препарат цитозинарабинозид, используемых на животных моделях, показало значительное увеличение продолжительности жизни мышей с лейкемией L1210 [50]. Применяя липосомы, достаточная доза активной формы лекарства может быть доставлена к целевому сайту защищенным образом [42].
Повышение специфичности и чувствительности липосомальных НЧ для терапевтического использования
Как упоминалось ранее, использование различных молекул и полимеров позволяет изменять структуру и мембрану липосом, и посредством этого к липосомам могут быть добавлены новые функциональные возможности или их свойства могут быть изменены [51]. Как продление циркуляции липосом в крови, так и увеличение их способности накапливаться в конкретной опухолевой ткани или патологическом участке за счет эффекта ЭПР являются первыми важными особенностями, которые следует принимать во внимание из-за высокой скорости клиренса липосом. Конъюгация молекул ПЭГ с липосомными мембранами посредством химической конъюгации последовательно использовалась для добавления этой функциональности к липосомам [52]. Важность и роль полимеров этиленгликоля в увеличении периода полужизни липосом, особенно липосомальных терапевтических НЧ в биологических жидкостях, таких как кровь, были выражены около 20 лет назад [53].
Abuchowski и McCoy предприняли первые попытки продлить период полужизни липосом в кровотоке путем конъюгирования PEG с их структурой. В результате их усилия в целом увеличили время циркуляции липосом и их период полужизни в кровотоке [54]. Через несколько месяцев другие исследователи исследовали возможность снижения высокой скорости клиренса липосом клетками системы мононуклеарного фагоцитоза (MPS). Ожидается, что за счет присоединения ПЭГ к поверхностным молекулам липосом [53] циркуляция липосом в крови улучшится. В этой области есть множество статей. Более того, в отличие от обычных липосом, липосомы, покрытые ПЭГ, демонстрируют дозозависимые фармакодинамические свойства [55]. Среди различных полимеров молекулы ПЭГ являются одними из полимеров, которые могут быть прикреплены к поверхности липосом для продления срока их хранения in vivo. Для этой цели можно использовать и другие полимеры [56]. На рисунке 5 показано, как молекулы полимера ПЭГ могут защищать липосомы от антител, а также продлевать их жизнь в кровотоке.
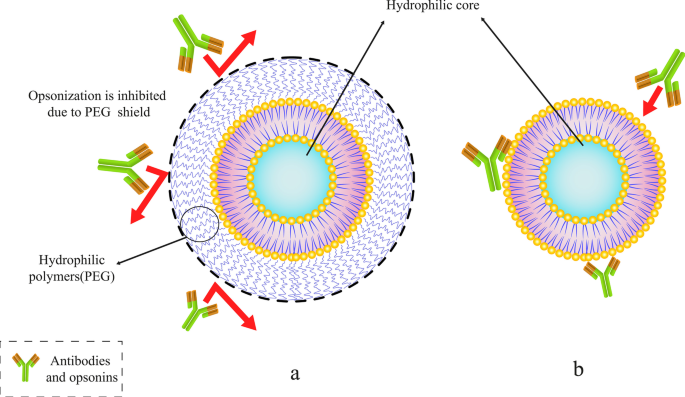
Конъюгирование определенного полимера, такого как полиэтиленгликоль (ПЭГ), с липосомами. а ПЭГилированные липосомы с молекулами полимера ПЭГ экранируют. б Обычные липосомы, захваченные антителами и опсонинами
Как уже упоминалось, примечательно, что другие молекулы могут использоваться для продления циркуляции липосом в дополнение к PEG. Кроме того, полимеры полиоксазолинов входят в число веществ, используемых для модификации липосомной мембраны с целью увеличения их периода полужизни. В связи с этим Woodle et al. были первой группой, применившей поли [2-этил-2-оксазолин] (PEOZ) для синтеза скрытых липосом. Их результаты свидетельствовали о снижении элиминации и поглощения поли [2-этил-2-оксазолилированных] петоксилированных липосом, вводимых крысам клетками печени и селезенки [57]. Их результаты показали, что конъюгация других полимеров, таких как поли [2-этил-2-оксазолин] и поли [2-метил-2-оксазолин] (PMOZ), может иметь PEG-подобные эффекты в увеличении периода полужизни и продлении циркуляция липосом in vivo. Они также сравнили биораспределение липосом, конъюгированных с PEG, PEOZ и PMOZ, в различных органах и системах. Последствия также показали, что биораспределение всех этих липосом в крови и селезенке было почти одинаковым, но в печени распределение PMOZ было намного ниже, чем у других [57].
Pain et al. связали молекулы декстрана с поверхностью УМО. Их результаты показали, что липосомы, конъюгированные с декстраном, по сравнению с обычными липосомами, имеют более расширенное кровообращение и более низкую абсорбцию и поглощение печенью и селезенкой. Это следствие свидетельствует о том, что молекулы декстрана, помимо продления срока хранения липосом в организме, также могут применяться для повышения стабильности и регулирования скорости высвобождения лекарственного средства из липосом [58].
Вторая проблема, на которую следует обратить внимание, - это текучесть и стабильность липосом. Другие липиды, включая холестерин, можно использовать в составе липосом. Иногда холестерин может замещать некоторые соединения в фосфолипидном бислое для усиления некоторых свойств липосом. Тем не менее, было доказано, что изменение содержания липосомного бислоя и замена некоторых молекул фосфолипидов определенными соединениями, особенно холестерином, может снизить текучесть липосом [59]. Кроме того, наличие холестерина в мембране липосом увеличивает стабильность их структуры (как в экспериментах in vivo, так и in vitro). Это также снижает проницаемость и возможность утечки захваченных веществ. Холестерин - это гидрофобный стероид, который взаимодействует с гидрофобными цепями между бислоями фосфолипидов, чтобы стабилизировать его структуру, когда он присутствует в мембране липосом. Это действие холестерина существенно при использовании липосом в клинических условиях in vivo, поскольку оно предотвращает превращение липосом в липопротеины высокой плотности (ЛПВП) и липопротеины низкой плотности (ЛПНП) в организме. Более того, липидные структуры, присутствующие в крови и внутриклеточных жидкостях, могут иметь отпечаток на липосомах. Липопротеины, такие как ЛПНП и ЛПВП, влияют на введенные липосомы и вызывают перенос липидов и перестройку их мембран. Они также резко снижают стабильность липосомальных НЧ, содержащих лекарственное средство [12]. Примечательно, что другие материалы, такие как ДНК и другие молекулы, используемые в липосомной мембране для терапевтического применения, должны быть прикреплены к холестерину в мембране. Добавление различных веществ к липосомным мембранам - один из способов придать липосомам положительные свойства [42].
Третьими важными характеристиками, которые следует учитывать, являются чувствительность и специфичность липосом для точной идентификации и специфического связывания с клетками-мишенями. Связывая соединения, такие как моноклональные антитела, F ab фрагментов и других конъюгированных молекул, таких как трансферрин и фолат, можно повысить специфичность липосом, что приведет к специфическому связыванию с опухолевыми клетками [60]. Кроме того, ранее исследовалось повышение специфичности и чувствительности наноносителей лекарств, в частности липосом. Например, Mohammad J. Akbar et al. изучили липосомы, конъюгированные с пептидом и ПЭГ-липидом, для лечения мелкоклеточного рака легкого (SCLC). Их результаты показали, что связывание пептида-антагониста рецептора гастрин-высвобождающего пептида (GRPR) с липосомами может увеличить специфичность и накопление этих липосом в GRPR-экспрессирующих клетках. Они также заявили, что эти липосомы, прикрепленные к пептидам, могут применяться для лечения клеток рака легких из-за активации в них генов, экспрессирующих GRPR [61].
В конце концов, лекарства и лекарства были добавлены к ПЭГилированным липосомам из-за их соответствующих свойств, и теперь эти липосомальные структуры были созданы для промышленного и клинического использования [62]. Антитела также использовались в ранних исследованиях для увеличения способности липосом связываться с клетками-мишенями [63]. В этом случае рецептор-опосредованный эндоцитоз осуществлялся липосомами, попадающими в клетку [64]. Между тем были разработаны различные методы связывания антител с липосомами [65]. Исследования липосом, конъюгированных с антителами, доказали, что токсичность противоопухолевых препаратов против культивируемых опухолевых клеток увеличивается при конъюгировании антител с поверхностями липосом [66]. Когда антитела наносили на поверхность липосом PEG, остаток антител для прикрепления к их рецепторам-мишеням был скрыт полимерами PEG, особенно когда боковые цепи, прикрепленные к PEG, были длинными [67]. Поэтому одновременное использование ПЭГ и антител для липосомных препаратов и его недостатки должны быть учтены учеными.
Четвертым важным фактором терапевтического применения липосом является этюд высвобождения содержащихся в них лекарственных средств. Регулировка липосом для извлечения внутри них лекарств, подверженных аномальному состоянию поврежденных тканей, является одним из важнейших вопросов при клиническом применении липосом. Кроме того, использование чувствительных к температуре соединений, pH или определенного метаболита на поверхности липосом, который может связываться с тканью и поверхностью мембраны клеток-мишеней, является методом точного высвобождения этих лекарств. Utilizing this method can result in the specific effect of liposomes on the membrane surface of target cells and also release the drug content inside them [68].
The releasing rapidity of compounds entrapped in liposomal NPs is the fifth substantial criterion for adjusting the dose of drugs available at the target site. One of the essential objects that should be considered for the proper usage of all kinds of drug delivery systems, including liposomes, is the releasing rate of drugs and regulation. With regard to liposomal drug delivery systems and NPs, it is worth mentioning that the encapsulated substances in the liposomes are not biologically available and can only be bioavailable while it is released from the initial state. Therefore, drug-containing liposomes can provide the ability to increase the concentration of bioavailable drugs for cancerous tissues and to improve the quality of treatment and therapeutic efficacy can be achieved on condition that the rate of drug release from the liposome is adjusted [69]. Furthermore, it has been proven that changing the liposome bilayer content and replacing some phospholipids with certain compounds, especially steroid molecules like cholesterol, can decrease the permeability and unintended leakage of the compounds stored in them [70]. Consequently, this advantage can be exploited to adjust the release rate of the encapsulated compound. Once released, the drugs must penetrate sufficiently into the cell and make the necessary physiological-biochemical changes to exert their impact.
As it is mentioned earlier, various compounds, including aptamers, can be conjugated to liposomes. In this regard, Mohammad Mashreghi et al. applied anti-epithelial cell adhesion molecule (anti-EpCAM) as an aptamer to functionalize Caelyx® liposomes. Their experiment outcomes determined that functionalization of Caelyx® with this aptamer could enhance the merits of this liposomal drug and made it a viable option for cancer treatment [71]. Figure 6 shows the structure of different types of liposomes that are used in vitro or for clinically scientific purposes schematically.
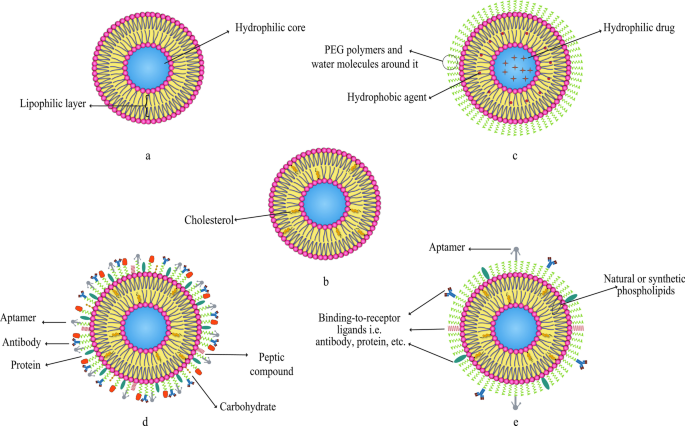
Various kinds of liposomes. а Conventional liposome; б cholesterol-conjugated liposome; c PEGylated or stealth liposome; г ligand-targeted liposome; е multi-functional liposome
The passage of drugs through lysosomes to enter cells (which have low pH and many degrading enzymes) is the sixth most important factor for the practical application of conjugated medicines to liposomes. To protect therapeutic agents from unwanted conversions in extracellular and intracellular space, cell-penetrating peptides are attached to the liposome surface [72].
On Liposomal Drugs Pharmacology:Pharmacokinetics and Pharmacodynamics
The assessment of pharmacological attributes, as an essential part of medicine and pharmaceutical science, is required not only to gain a better understanding of liposomes pros and cons as drug carriers but also to confirm and evaluate them in clinical trials. The pharmacological properties of liposomal drugs and their interactions with the body can be examined in two various aspects:pharmacokinetic (the effect of the body on therapeutic compounds) and pharmacodynamics (how medications act and impact the body and cellular pathways) [73]. In general, the utilization of liposomes for drug delivery in cancer treatment or other disorders requires the elevation of these agents' effectiveness on the one hand, and reducing their toxicity toward normal tissues on the other hand. Subjects such as the proper administration route of NP-based drugs, their circulation in the bloodstream and half-life, their biological distribution in tissues, and their cellular metabolism, as well as their elimination, metabolization and clearance, have been studied in the field of pharmacokinetics [74]. The pharmacokinetics of liposomes primarily study the bioavailability of liposome-conjugated drugs in various body fluids and tissues. Indeed, the study of chemical decomposition and biological excretion, and liposome uptake and purification are also considered in pharmacokinetics. The results of studies on pharmacological advantages of using liposomal drugs (regardless of the type of liposomes applied in the DDS) instead of free drugs showed that:
Primarily, liposome can modify the drug release profile to a sustained release, and consequently, reduce the requirement for constant injection. Secondly, it can extend the presence of the drug in the bloodstream and body fluids, and as a consequence, increase its half-life. Thirdly, it has the potential to lead to better bio-distribution in cancerous tissues while reducing drug influences on healthy tissues due to limited particle size to cross the Endothelium of healthy capillaries. Ultimately, it reduces drug metabolism and inactivation in plasma before reaching the target tissue, in addition to its positive effect on the clearance of drug metabolites [75, 76].
However, some changes are required in the pharmacokinetics of liposomes to increase their solubility, specificity, and sensitivity. These modifications enable them to overcome chemotherapy-resistant cells, enhance the efficacy and half-life. Moreover, their toxicity or unintended metabolic compounds product as a result of their metabolization should be decreased by these modifications [77].
After consuming liposomal drugs administration, they enter the body and circulate in the bloodstream with a specific half-life. Their size and formative composition determine the half-life of liposomal medications. Moreover, rapid clearance of liposomal drugs from the body can reduce their duration of action and therapeutic index. As aforesaid, appending hydrophilic polymers such as PEG to liposomes is able to decrease their clearance rate and solve this challenge [78]. Also, it is possible to adjust the fluidity and drug-release rate of the liposome membrane by adding cholesterol molecules.
The application of liposomes for drug delivery may lead to some changes in drug pharmacokinetics [79]. The ability of liposomes to change the pharmacokinetic properties of the various drugs and medications is one of their significant benefits in drug delivery systems [80]. Concerning the process of liposome clearance and elimination, it is obvious that liposomal structures are affected by plasma proteins after being administered. For instance, after injection of liposomal nanoparticles opsonins are adsorbed on the surface of the liposomes. Opsonins are plasma protiens which mostly include immunoglobulins and fibronectin [42]. Opsonins presence on the surface of liposomes will result in their elimination by MPS, as one of the significant elimination section of various drugs from blood and body fluids. They also clear liposomes through the attachment of some receptors such as complement C3b and Fc to opsonins-liposomes complex [81]. Various tissues and cells such as liver kupffer cells, macrophages present in the spleen, bone marrow, and lymph nodes are involved in the clearance of liposomal NPs [82].
According to the International Union of Pure and Applied Chemistry (IUPAC) definition, pharmacodynamics refers to the study of the pharmacological impact of compounds on living systems and the biochemical and physiological consequences of these effects [83]. The increased elusion to identify therapeutic agents when encapsulated in liposomes has been recognized as one of the pharmacodynamical benefits of liposomes utilization [84].
Furthermore, the physicochemical characteristics have significant influences on the pharmacology of liposomal drugs. The particle size, electrical charge of membrane, and the composition of membrane lipids are some of these physicochemical properties that can affect the pharmacokinetics and pharmacodynamics of the agents. Firstly, there is a direct relationship between the particle size of nanoparticles, including liposomes, and their clearance rate. By increasing the size of NPs, their elimination rate by the immune system and MPS cells will also enhance [85]. Secondly, it is worth mentioning that the net charge of liposome membranes is a consequence of the electrical charge of phospholipids and their other constituting particles that made them up. As a result, a rise in the membrane charge is associated with enhanced clearance rates of these agents [86]. The composition of membrane lipids and other structural features (such as hydrophilic core radius) also remarkably affect the pharmacokinetics of liposomal drugs [87].
More importantly, it has been hypothesized that different types of liposomes exhibit distinct drug kinetics/dynamics depending on their various structures. The drug release rate also rests on the number of phospholipid bilayers and the content of loaded drug compounds. It is also contingent upon the hydrodynamic diameter, total volume, and other pharmacokinetic properties as well [88].
Administration Route of Liposomal Drugs
Like many different drugs, NP-based liposomal medicines can be administered from a wide variety of routes. In other words, oral consumption [89] and distinct injection methods such as intravenous (I.V.) administration and various local injections are among the common administration routes of liposomal drugs [90]. The usage of nanoparticles, including liposomes, for drug delivery via oral administration has been highlighted as an effective strategy since the nanoparticles increase the bioavailability of medicines, improve their interaction with cells, and prevent any modifications in the molecular structure of the drug due to enzymes and gastric juices in the gastrointestinal tract. Moreover, they have the ability not only to enhance the release of remedial molecules into the mucosal and epidermal layer but also to protect drugs from unwanted changes during the first pass effect [89]. Intravenous injection is used as the primary administration route for many liposomal drugs approved by the FDA or other authorities [42]. On the other hand, subcutaneous (S.C.), intradermal (I.D.), intraperitoneal (I.P.), and intramuscular (I.M.), classified under the title of the local injection, are also utilized for administration of liposomal drugs [90,91,92].
Liposomal Drugs Fate In Vivo and Their Targeting Mechanism of Action
Following administration of the liposomal drugs, they reach the pathological lesions at the target site through the bloodstream and accumulate there. The mechanism of action of liposomal drugs on tumors starts with their accumulation at the target site, uptake of them by tumor cells, and the release of free drugs [93]. Subsequent to entering the body, liposomal drugs reach the tumors through various targeting mechanisms of action and then interact with cells in different ways [94]. In general, tumor-targeting mechanisms are divided into two categories:passive and active targeting. Passive targeting refers to the mechanisms in which liposomes are spontaneously accumulated at the tumor site and interact with target cells without the presence of a specific ligand [95]. The effect of enhanced permeability and retention (EPR) has been suggested as the most critical passive targeting mechanism. To be precise, the spontaneous accumulation of therapeutic NPs and liposomal drugs at the tumor site is called the EPR effect [96]. This phenomenon can be assigned to the leaky nature of tumor tissue vessels, unlike normal tissue capillaries, which makes them permeable to molecules and NPs. Consequently, This ultimately leads to the accumulation of drug compounds in these tissues and the effect of EPR [97]. The ultimate fate of the drug in the intracellular fluid and cytoplasm of tumor cells depends on several factors such as release mechanism, nanocarrier constituents, and molecule structure [98]. In healthy tissue, the number and the shape of capillaries are proportionate and normal, respectively. However, in cancerous organs, unlike healthy tissue, the number and the structure of capillaries are higher and deformed, respectively, because of the angiogenesis process. Moreover, the tumor capillaries structure is destroyed, and the endothelial phalanx cells are diminished. As a result, the volume of plasma fluid leaking into the intercellular space will be enhanced. In healthy tissue, however, capillary phalanx cells retain cellular tight adhesions, preventing NPs, small molecules, and liposomal drugs from seeping into the intercellular space [99]. The EPR effect in cancerous capillaries and their difference with normal and healthy tissue vessels are illustrated in Fig. 7.
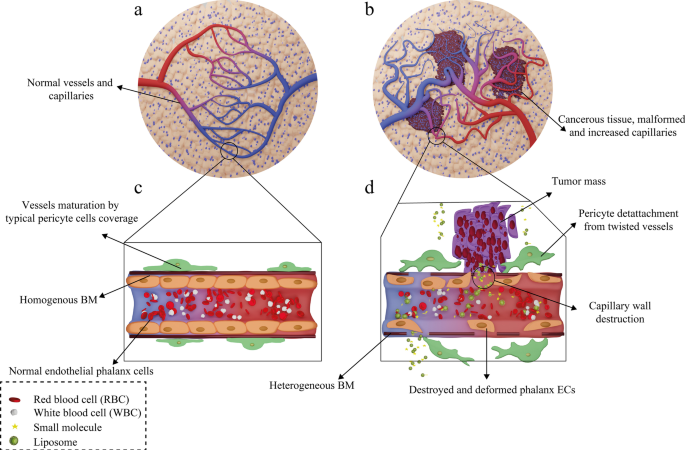
Mechanism of action of the drug-containing liposomes on tumor cells via EPR effect. а Healthy tissue and its normal capillaries; б cancerous tissue with increased-deformed vessels; c structure of normal and healthy vessel; г destructions and deformed capillary in tumor tissue
On the other hand, active targeting has attracted considerable attention as one of the targeting mechanisms of action owing to its appropriate effectiveness and high specificity. Active targeting includes various types and is also generally aimed to reduce the off-target impacts of liposomal NPs on healthy cells and non-target tissues [95]. In this method, molecules such as monoclonal antibodies, small molecules, signal peptides, vitamins, particular carbohydrates, glycolipids, or aptamers are generally utilized for surface modification of liposomes [100, 101]. Moreover, active targeting can be split into various subtypes according to diverse features. For instance, it can be classified into two general categories:
- 1.
Targeting tumor cell and cancer tissue receptors:This method relies on conjugating specific molecules to the membrane surface of liposomes, making them able to bind to special or overexpressed receptors on cancer cells [102]. In cancer cells, upregulation of different genes causes an increase in the expression of specific cell surface receptors in response to enhanced metabolic demands for rapid cell proliferation [103]. In active targeting, particular molecular modifications can be applied for targeting specifically the overexpressed surface receptors of cancer cells, such as folate receptor (FR), transferrin receptor (TfR), or Epidermal growth factor receptor (EGFR) [95]. In this regard, the role of folate receptors in cancer cells is to increase folic acid uptake [104], whereas transferrin receptors bind to transferrin (as a free molecule with 80 kDa weight in serum) and cause endocytosis of this monomeric glycoprotein to occur [105]. Moreover, EGFR receptors are a class of tyrosine kinases involved in cellular processes such as tissue differentiation and repair. The expression of this receptor in cancer cells is significantly increased due to its involvement in processes such as angiogenesis, cell proliferation, and metastasis [106].
- 2.
Utilizing tumor microenvironment as the target:In this method, changes in the surface of liposomes are exploited to enable them to target signal peptides or other receptors in the microenvironment of cancer cells. In other words, this active targeting mechanism can inhibit the growth of tumor cells and metastasis, prevent genotypic and phenotypic variations in neovascular endothelial cells, and control drug resistance [107]. Furthermore, some receptors in the tumor microenvironment, such as Vascular endothelial growth factor (VEGF), Vascular cell adhesion protein (VCAM), matrix metalloproteases, and integrin, are targeted in this mechanism [95].
Cellular Uptake of Therapeutic NPs and the Effect of Liposomal Drugs on Targeted Cells:Actions and Interactions
As it is mentioned earlier, liposomes are able to target tumor cells either passively or actively. After the liposome reaches the cancerous cells and the tumor environment through the targeting mechanism, it can release its therapeutic content and exert its effects by means of various mechanisms. Consequently, lipid composition, the surface charge of the membrane, type of cancer, type of target cells, as well as the presence of specific ligands on the liposome membrane, can influence the cell-liposome interaction [108].
Figure 8 illustrates different types of liposomes interactions with target cells. After being injected into the body, drug-containing liposomes travel to different tissues through blood vessels and eventually reach their target cells based on their surface ligands. These liposomes can bind to cellular receptors via these ligands, which is called specific absorption [42]. Albeit, receptor-free liposomes can also adhere to the target cell surface through molecular attractions, electrostatic forces, and molecular interactions called non-specific absorption. Following liposomes binding to the cell, the therapeutic agent is released into the cytoplasm, and its effects may be produced in different ways. The liposomal nanocarriers can be entirely fused to the plasma membrane of the cell and release the drug. Drug compounds are also able to be released from the liposome into the cell and to enter the cell through micropinocytosis or passive diffusion without the occurrence of fusion. Liposomes may directly interact with the cell or exchange lipid fragments with the cell membrane through protein-mediated processes. At the same time, the drug may act on the cell and exert the therapeutic effects of the liposomal drug. However, some liposomes are capable of entering through endocytosis (specific or nonspecific). In particular, liposomes penetrating the cell via this passage can have various destinies. It is possible for them to combine with lysosomes. In such cases, lysosomal enzymes affect the structure of the drug by reducing the pH of the phagolysosome sac. Ultimately, liposomes release the drug by fusing it to the cell membrane or endocytosis, and after that, medications exert their therapeutic effect [42, 62]. All possible ways for the liposome to penetrate the cell and exert its effect are depicted and compared in Fig. 8.
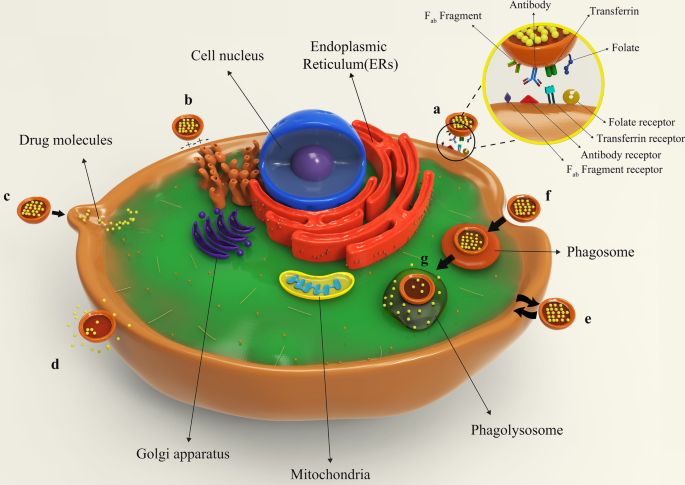
Binding of liposomes to the target cell. а Specific attachment via ligand-receptor interaction; б non-specific absorption of liposomes through intramolecular-electrostatic forces; c the attachment and fusion of liposome to the cell membrane and drug release; г liposome arrival to the target cell and drug release without fusion; е exchange lipid fragments between the cell membrane and liposome through protein-mediated processes; е endocytosis of liposome by target cell; г lysosomal digestion of liposome in the cell cytoplasm
On the other hand, NP-based medications can undergo endocytosis, pinocytosis, or phagocytosis by the target cells. Endocytosis is known as the process in which compounds outside the cell space approach the cell membrane and then enter the cell as a vesicle [109]. Pinocytosis, also recognized as fluid endocytosis, occurs when small molecules or suspensions are introduced into a cell through a vesicle by creating an invagination in the cell membrane. Moreover, pinocytosis vastly occurs in human cells to absorb fat droplets. In an immunological study, Yuriko Tanaka et al. reported that liposome-coupled antigens pinocytosis can be performed by antigen processing cells (APC). This report had proved that liposomes can undergo pinocytosis mechanisms [110]. In phagocytosis, particles larger than 0.5 μm are engulfed by immune cells, it may also occur for liposomes (especially for MLVs and liposomes larger than 500 nm). For example, Jitendra N. Verma et al. confirmed the occurrence of phagocytosis on liposomes by a study on the phagocytosis of liposomes with malarial antigens by macrophages [111].
Kaposi's Sarcoma, One Instance of Successful Liposomal Drugs Applications
Kaposi's sarcoma is a progressive multifocal anti-proliferative cancer primarily known as endometrial sarcoma. This cancer is more common in HIV patients whose immune system is weakened. Furthermore, it has been commonly seen in skin tissue and may also involve other tissues. Hence, this disorder is generally referred to as skin mucosal sarcoma [112]. To treat this disease, modified long-circulating liposomes can be helpful. In this regard, liposomes passively target tumor cells. Moreover, the effect of EPR and specific binding increases the concentration of the therapeutic drug in cancer tissues 5 to 11 times higher than normal skin [113]. For this purpose, Doxorubicin is used for the treatment of this disease. Correspondingly, entrapment of the doxorubicin into liposomes (which was PEGylated to prolong its half-life) prevents normal tissues from being exposed to the drug. It also reduces drug uptake by these healthy doxorubicin-sensitive tissues such as the heart [114].
Additionally, the liposomal form of doxorubicin, Doxil, is a type of anthracycline drug which is approved for clinical administration by US-FDA. It is used to treat AIDS-related Kaposi sarcoma and multiple myeloma [115]. Doxil has better therapeutic efficacy and less toxicity than free doxorubicin, which can be attributed to its ability to target tumors indirectly. It is also passive targeting due to leakage of tumor vessels and the EPR effect [116]. Moreover, the Doxil unilamellar liposomes are < 100 nm in size and have been used to treat various cancer types [42]. Analyses have also proved that free doxorubicin concentration is lower than that of Doxil at the target tissue site [117]. In this regard, Ogawara et al. investigated the effect of Doxil (formed by binding doxorubicin to PEG liposomes) on cancer cells in male mice and showed that PEG liposomal doxorubicin or Doxil1 had been effective on both doxorubicin-resistant and doxorubicin-sensitive C26 cell groups [118]. This can highlight the significance of the exploitation of liposomal NPs. Because they can be consumed to overcome the resistance of cancer cells to common chemotherapy agents at low costs without time-consuming research works to discover new clinical therapeutic compounds [119]. The application of nanoparticles, such as liposomes, to deliver doxorubicin to tumor tissues has been widely investigated. Entrapment of ATP-binding cassette transporter superfamily B member 1 (ABCB1) substrate doxorubicin into liposomes can increase drug uptake and enhance its intracellular distribution within cancer cells, especially ABCB1-expressing cancer cells [120]. The simple structure of Doxil is illustrated in Fig. 9.
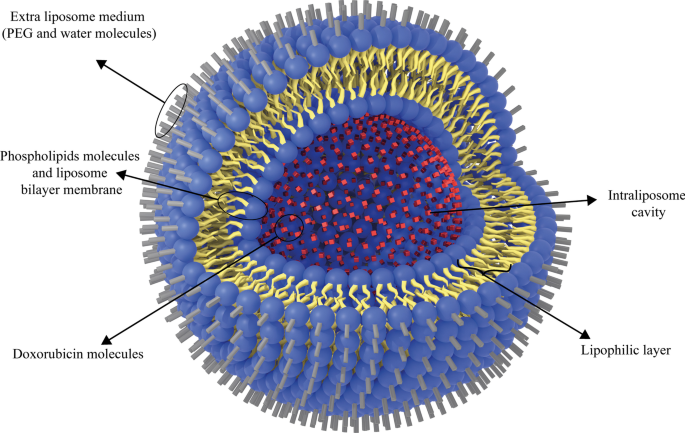
The schematic structure of Doxil drug. Doxorubicin drug molecules are entrapped in the hydrophilic cavity of unilamellar PEGylated liposomes
Furthermore, liposomal nanomaterials can be exploited for the treatment of infectious diseases. Systemic fungal infection is one of the most challenging conditions that is usually treated with amphotericin B, which is highly toxic to kidney cells. For this purpose, the usage of liposome-entrapped amphotericin B can reduce the toxicity of this drug compared to its free form [48]. Unilamellar liposomes have been used to entrap this agent. It has proven that liposomal amphotericin B is more effective than the free drug form [121]. Based on the formulation, these liposomes also alter the bio-distribution of amphotericin B, such as anticancer drugs, which in turn not only arrange the mechanism of action but also increase the effective dosage concentration at the target tissue [122]. AmbiSome, liposomal form amphotericin B, is approved for public administration too. Other approved liposomal drugs, from anti-fungal medications to cancer therapeutic agents, are summarized in Table 1.
Although the application of liposomal NPs to treat cancer has been touted as a viable solution for drug delivery and affecting tumor cells, drug delivery to cancerous tissues in the central nervous system (CNS) has remained a significant challenge. In addition, drug delivery to central nervous system cells faces many turbulences owing to a blood–brain barrier (BBB). However, this problem can be partially solved by developing new methods and using lipid-based compounds [136].
Liposomal Nanoparticles in the Investigational Phase for Therapeutic Purposes
Liposomal siRNA
RNA is a type of genetic molecule with a variety of functions, including translation and transcription processes. The discovery of small-interfering RNA (siRNA) is a significant advance in biology in the last decade [137]. Synthetic siRNAs can be utilized to target oncogenes and their mRNAs. Furthermore, siRNAs can be applied for targeting genes contributing to the carcinogenesis, proliferation, and metastasis of tumor cells or their resistance to standard chemotherapies and radiation [138]. Therefore, it has been considered a modern method for cancer therapy. On the other hand, the nanoparticles used to deliver siRNA must possess properties such as biodegradability, great bio-distribution, low toxicity, etc. All of these features can be offered by liposomes making this popular drug delivery system a promising candidate for this purpose [28]. siRNAs bound to neutral lipid-based NPs are well isolated from these liposomes. They also influence ephrin type-A receptor 2 (EphA2), focal adhesion kinase (FAK), neuropilin-2, Interleukin 8 (IL-8), and TROJAN Mobile Remote Receiving System/erythroblast transformation-specific (TMRRS/ERG), Elongation factor 2 kinase (EF2K) or Bcl-2 pathways. Following the occurrence of this mechanism, a suitable antitumor effect has been observed against ovarian, colon, and breast cancer cells, etc. [139, 140]. Numerous studies have been conducted on siRNA delivery by liposomes, and in most of them, the cationic lipid Dioleoyl-3-trimethylammonium propane (DOTAP) has been widely expended in the structure of liposomes. Due to DOTAP high positive charge, this cationic lipid can be toxic to cells. It can stimulate cellular hemolysis and reduce ultimate biocompatibility as well. This has challenged the application of this lipid in the composition of liposomes applied for siRNA delivery [141].
Liposomal Curcumin Nanoparticles
Curcumin-conjugated liposomes are another instance of liposomal nanoparticle usage. Curcumin is a natural polyphenolic and hydrophilic compound that is abundant in the Curcuma longa plant and can be mainly prepared from turmeric extraction. Nowadays, the anticancer effect of curcumin has been well indicated against many tumor cells, such as breast cancer, liver carcinoma, and prostate cancer, etc. [142]. The primary mechanism of action of curcumin against cancer cells is to interfere with the translation of proteins such as Bcl-xl and regulate apoptosis by influencing their process, controlling the release of reactive oxygen species (ROS) and cytochrome, regulating molecular factors such as cyclin affecting the cell cycle. On the other hand, curcumin can damage the nuclear and mitochondrial DNA structure of liver cancer cells, thereby disrupting their function [143]. In comparison with free curcumin, the application of liposomal curcumin improves pharmacokinetics and pharmacodynamics while reducing the dosage required to target tumors. Matheus Andrade Chave et al. explored curcumin-containing liposomes by inserting curcumin molecules into the MLV liposome [144]. The synthesis of liposomal curcumin and curcumin structure are described in Fig. 10.
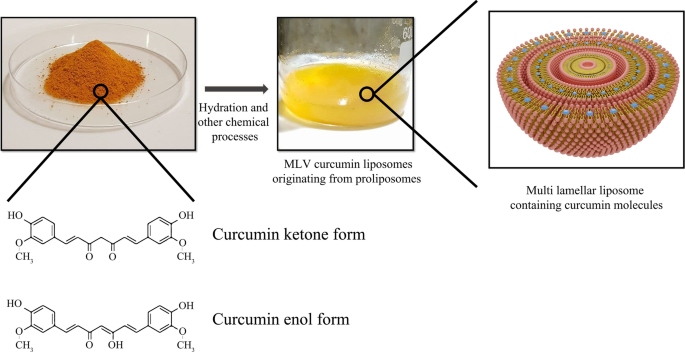
An overview of curcumin powder and liposomal curcumin synthesis. Chemical reactions performed for liposomal curcumin production and curcumin molecule structure in various forms are simply demonstrated
In addition, liposomes prepared for therapeutic research applications can be synthesized by employing various methods. For example, Qiao Wang et al. exploited the ultrasonication and lipid film-hydration method to synthesize daidzein long-circulating liposomes (DLCL) [145]. Xiaoyuan Ding et al. also used the film hydration method for the synthesis of aptamer and Au-NPs (Apt-Au)-modified Morin pH-sensitive liposome. Their outcomes showed high biocompatibility and insignificant toxicity of these liposomal structures and highlighted these liposomes as a viable option for selective targeting of tumors [146].
Other Liposomal NPs in the Investigational Phase
Several liposomal drugs have been synthesized and utilized in various medications at the investigational phase. For instance, CPX-1 was produced by entrapping the antitumor agents, Irinotecan and floxuridine (1:1 molar ratio) in liposomes, and was designed to treat advanced colorectal cancer. This therapeutic nanoparticle is in phase II research status [128]. Lipovaxin-MM is another momentous liposomal nanoparticle in phase I research prepared by placing melanoma antigens in liposomes and mainly administrated for immunotherapy of malignant melanoma. This agent is also under investigation [128].
Conclusion
As spherical structures in liquids, liposomes can be applied as a promising option for cancer therapy and drug delivery, as well as imaging, and disease management. By reviewing liposomes pros and cons, scientists will be able to improve them in future research works.
Some opportunities and challenges in liposomes utilization are described in the following. One of the convenient features of liposomes is their morphological similarity to cells (presence of phospholipids), as well as increasing the effectiveness of the drugs. As a negative point, liposomal phospholipids may sometimes undergo hydrolysis or oxidation reactions which may be problematic. Other pros of liposomes include increased stability of the encapsulated drug in it, reduced contact of sensitive tissues with therapeutic molecules, decreased drug toxicity, improved pharmacokinetic and pharmacodynamics properties, the ability to regulate the rate of drug release, and the potential of their structure to accept the desired chemical modification. In contrast to these opportunities, there are some challenges such as leakage or unintended entrapment of drugs, low liposome bioactivity, decreased-solubility, rapid clearance of conventional liposomes from the blood by the reticuloendothelial system (RES), and problems caused by continuous intravenous administration or local injection.
Besides examining the advantages and disadvantages of liposomes, we should take their proper targeting mechanism of action into account. Passive targeting is considered a beneficial mechanism due to the abundant clinical evidence and experience. It also increases the circulation time of liposomal drugs. The problem of this mechanism lies in its non-specific drug delivery and its physiological barriers. In contrast, beneficial features of active targeting include increased specificity in drug delivery, the possibility of overcoming chemotherapy-resistant tumor cells, and reduced off-target effects. However, the difficulty in identifying accurate binding sites on cancer cells and the lack of adequate evidence of its former utilization have led to some ups and downs in its application.
Liposomes are reasonable candidates for elevating the effectiveness of current anticancer agents and preventing the incidence of drug resistance. Future research in this area should be focused on further investigation into the properties of liposomal structures. To probe about drug entrapment in therapeutic nanoparticles, including liposomes, much more detailed examinations will be required.
Сокращения
- WHO:
-
World Health Organization
- VNPs:
-
Viral nanoparticles
- НП:
-
Наночастицы
- US FDA:
-
United States Food and Drug Administration
- DDSs:
-
Drug delivery systems
- ПК:
-
Phosphatidylcholine
- SM:
-
Sphingomyelin
- PS:
-
Phosphatidylserine
- PE:
-
Phosphatidylethanolamine
- ULVs:
-
Unilamellar vesicles
- OLVs:
-
Oligo lamellar vesicles
- MLVs:
-
Multilamellar vesicles
- SUVs:
-
Small unilamellar vesicles
- RBF:
-
Round-bottom flask
- LNs:
-
Liposomal nanoparticles
- MPS:
-
Mononuclear phagocytosis system
- PEG:
-
Полиэтиленгликоль
- PEOZ:
-
Poly [2-ethyl 2-oxazoline]
- PETOXylated:
-
Poly [2-ethyl-2-oxazolylated
- PMOZ:
-
Poly [2-methyloxazoline]
- HDL:
-
High-density lipoprotein
- LDL:
-
Low-density lipoprotein
- SCLC:
-
Small cell lung cancer
- GRPR:
-
Gastrin-releasing peptide receptor
- Anti-EpCAM:
-
Anti-epithelial cell adhesion molecule
- IUPAC:
-
International Union of Pure and Applied Chemistry
- I.V.:
-
Intravenous
- S.C.:
-
Subcutaneous
- I.D.:
-
Intradermal
- I.P.:
-
Intraperitoneal
- I.M.:
-
Intramuscular
- EPR:
-
Enhanced permeability and retention
- fR:
-
Folate receptor
- TfR:
-
Transferrin receptor
- EGFR:
-
Epidermal growth factor receptor
- VEGF:
-
Vascular endothelial growth factor
- VCAM:
-
Vascular cell adhesion protein
- APC:
-
Antigen-presenting cells
- ABCB1:
-
ATP-binding cassette transporter superfamily B member 1
- HSPC:
-
Hydro soy phosphatidylcholine
- DSPG:
-
1,2-Distearoyl-sn-glycero-3-PG
- DOPC:
-
Dioleoylphosphatidylcholine
- DPPG:
-
1,2-Dipalmitoyl-sn-glycero-3-phosphoglycerol
- DSPC:
-
1,2-Distearoyl-sn-glycero-3-phosphocholine
- AML:
-
Acute myeloid leukemia
- ALL:
-
Acute lymphocytic leukemia
- DSPE:
-
1,2-Distearoyl-sn-glycero-3-phosphoethanolamine
- DOPE:
-
Dioleoylphosphatidylethanolamine;
- EPG:
-
Esterified propoxylated glycerols;
- DMPC:
-
1,2-Dimyristoyl-sn-glycero-3-phosphocholine
- DOPS:
-
1,2-Dioleoyl-sn-glycero-3-phospho-L-serine
- POPC:
-
1-Palmitoyl-2-oleoyl-sn-glycero-3-phosphocholine
- DMPG:
-
1,2-Dimyristoyl-sn-glycero-3-phosphoglycerol
- MPEG:
-
Methoxypolyethylene glycols.
- CNS:
-
Central nervous system
- BBB:
-
Blood–brain barrier
- siRNA:
-
Small-interfering RNA
- EphA2:
-
Ephrin type-A receptor 2
- FAK:
-
Focal adhesion kinase
- IL-8:
-
Interleukin-8
- TMRRS/ERG:
-
TMRRS/ERG TROJAN Mobile Remote Receiving System/erythroblast transformation-specific
- EF2K:
-
Elongation factor 2 kinase
- DOTAP:
-
Dioleoyl-3-trimethylammonium propane
- ROS:
-
Активные формы кислорода
- DLDC:
-
Daidzein long-circulating liposomes
- RES:
-
Reticuloendothelial system
Наноматериалы
- Правильный выбор насоса жизненно важен для обработки семян
- Нановолокна и нити для улучшенной доставки лекарств
- Наночастицы для терапии рака:текущий прогресс и проблемы
- Металлоорганические каркасы, реагирующие на окружающую среду, как система доставки лекарств для терапии опу…
- Доставка лекарств на основе клеток для онкологических приложений
- Рыба-данио:многообещающая модельная система в реальном времени для доставки нейроспецифических лекарств, оп…
- PLGA-липидные наночастицы с отслеживанием 131I как носители доставки лекарств для целевого химиотерапевтическо…
- УФ-обработка гибких сетчатых пленок из медных нанопроводов для применения в прозрачных проводниках
- Наноносители на основе нуклеозидов и липидов для доставки сорафениба
- Микророботы, напечатанные на 3D-принтере, обещают доставку лекарств