Многофункциональные наночастицы золота для улучшенных диагностических и терапевтических применений:обзор
Аннотация
Медицинские свойства металлов веками изучались в традиционной медицине для лечения инфекций и болезней и применяются до сих пор. Препараты на основе платины - это первый класс препаратов на основе металлов, которые будут клинически использоваться в качестве противоопухолевых средств после одобрения цисплатина Управлением по санитарному надзору за качеством пищевых продуктов и медикаментов США (FDA) более 40 лет назад. С тех пор для клинических испытаний было одобрено больше металлов, полезных для здоровья. Интересно, что, когда эти металлы редуцируются до металлических наночастиц, они проявляют уникальные и новые свойства, превосходящие их массивные аналоги. Золотые наночастицы (AuNP) входят в число металлических наночастиц, одобренных FDA, и показали большие перспективы в различных областях медицины. Они использовались в качестве средств доставки лекарств, фототермических (ФТ), контрастных, терапевтических, радиосенсибилизирующих агентов и агентов генной трансфекции. В данном документе рассматривается их биомедицинское применение, охватывающее их потенциальное использование в диагностике и лечении заболеваний. Также обсуждаются некоторые системы на основе AuNP, одобренные для клинических испытаний, а также потенциальные угрозы здоровью, связанные с AuNP, и некоторые стратегии, которые можно использовать для улучшения их биосовместимости. Рассмотренные исследования предлагают доказательство принципа того, что системы на основе AuNP потенциально могут использоваться отдельно или в сочетании с обычными системами для повышения их эффективности.
Введение
Медицина - одна из многих областей, в которых нанотехнологии выиграли. Нанотехнологии открыли множество возможностей для улучшения и разработки новых диагностических и терапевтических средств с использованием наноматериалов [1, 2]. В частности, AuNP обладают уникальными физико-химическими свойствами и хорошей химической стабильностью. Их легко функционализировать почти со всеми типами электронодонорных молекул, с помощью различных химических процессов или благодаря их сильному сродству к тиолированным молекулам [3, 4]. Из-за своего крошечного размера AuNP имеют большую площадь поверхности и высокую способность загружать лекарство. Множественные фрагменты могут быть включены в AuNP для биомедицинских приложений; к ним относятся нацеленные молекулы для повышения специфичности, контрастные агенты для биовизуализации и мониторинга реакции болезни на лекарства в режиме реального времени, а также терапевтические агенты для лечения болезни [5, 6]. Интересно, что даже без каких-либо добавленных биомолекул AuNP способны нацеливать, визуализировать и лечить заболевания. На основе их свойств, зависящих от размера, новые системы на основе AuNP могут быть созданы для использования в различных биомедицинских приложениях [7].
AuNP изготовлены из предшественника металла, который является термостабильным и, следовательно, очень стабильным и небиоразлагаемым. Массовое золото используется в медицине и доказало свою биоинертность и нетоксичность [8, 9]; следовательно, золотое ядро в AuNP будет по существу проявлять аналогичные свойства [3, 10]. AuNP и их применение широко изучаются на протяжении более пяти десятилетий и показали большие перспективы в качестве тераностических агентов в доклинических [5, 11,12,13] и клинических исследованиях [14,15,16,17,18]. Как описано в этом обзоре, существует гораздо больше возможностей для новых систем на основе AuNP. AuNP уже исследуются в клинических испытаниях в качестве носителей лекарств для лечения поздних стадий рака [16, 17], а также в качестве агентов ПК при лечении рака простаты [19] и угрей [18]. Без ущерба для здоровья и нормативных вопросов, связанных с использованием AuNP [20], будущее этих систем в биомедицине уже близко. Возможны многофункциональные системы на основе AuNP, которые способны бороться с лекарственной устойчивостью с локальной и повышенной эффективностью [11, 21, 22]. Обзор подчеркивает биологические свойства AuNP в доклинических и клинических исследованиях, размышляя об их биологическом применении в качестве диагностических и терапевтических агентов. Также описаны их потенциальные угрозы здоровью и стратегии, которые использовались для преодоления их ограничений. Наконец, освещены будущие перспективы AuNP в медицине.
Золотые наночастицы
Популярность AuNP в медицинских приложениях резко возросла благодаря их уникальным химическим и физическим свойствам. AuNP представляют собой твердые коллоидные частицы размером от 1 до 100 нм [23]. Применение AuNPs в биологии связано с их физико-химическими свойствами, не ограничиваясь их размером, поверхностным плазмонным резонансом (SPR), формой и химией поверхности [3, 10]. Эти параметры влияют на их активность и делают их идеальными кандидатами для использования в диагностике и лечении заболеваний в качестве средств доставки, сенсибилизации, контрастирования или терапевтических средств. Их небольшой размер связан с большей площадью поверхности, что позволяет модифицировать поверхность и прикреплять несколько полезных нагрузок, таких как нацеливание, визуализация и терапевтические агенты [4, 24, 25, 26]. Их небольшой размер также позволяет НЧ и их грузу преодолевать биологические барьеры, которые иначе трудно достичь и проникнуть [11].
AuNP все чаще признаются в качестве возможных диагностических, терапевтических и тераностических (агент, который может одновременно использоваться для диагностики и лечения заболевания) агентов, которые могут устранять нецелевые эффекты, связанные с традиционными методами лечения. Однако AuNP обладают другими свойствами и функциями по сравнению с их биосовместимыми массовыми аналогами, что может быть опасным для здоровья человека [27,28,29]. Клиническое использование нерасфасованных соединений золота для лечения заболеваний - древняя практика и сертифицирована как безопасная [8]. В последние годы исследования показали, что AuNP обладают схожими или улучшенными медицинскими свойствами [29]. Благодаря своим уникальным оптическим, химическим и физическим свойствам AuNP часто обладают новыми свойствами по сравнению с массивным золотом [30, 31] и могут служить диагностическими и терапевтическими средствами [5].
Синтез AuNP
AuNP могут быть созданы несколькими способами, используя либо восходящий, либо нисходящий подход. Нисходящий подход использует физические и химические методы для получения желаемых размеров из объемного материала, в то время как восходящий подход включает химические методы для сборки строительных блоков при формировании наноразмерных систем [32, 33]. Физические методы (такие как измельчение, фотохимия, облучение и литография) требуют значительных затрат энергии и давления, чтобы уменьшить объемные материалы до 10 –9 миллиардная метра размером [10, 32, 34]. Процессы нуклеации легко контролируются при использовании физических методов, восстанавливающие агенты не требуются, и с некоторыми из этих методов синтез происходит одновременно со стерилизацией НЧ. Однако физические технологии часто бывают дорогостоящими, труднодоступными и требуют специального оборудования. Более того, укупорочные и стабилизирующие агенты могут не выдержать высокоэнергетических процессов, связанных с этими процессами [34].
Восходящий подход наиболее предпочтителен при синтезе AuNP, поскольку он быстрый, простой и не требует использования сложного оборудования [33,34,35]. Он основан на химическом методе, разработанном Туркевичем в 1951 году (рис. 1A), который использует цитрат для восстановления и стабилизации предшественника золота, что приводит к получению 15-нанометровых сферических AuNPs [3, 10, 23, 33, 36 , 37]. Метод был дополнительно модифицирован путем варьирования соотношения содержания цитрата к содержанию прекурсора золота, что привело к диапазону диаметров AuNP 15–150 нм (рис. 1B) [10, 24]. Также был введен ряд восстановителей, таких как боргидрид натрия, бромид цетилтриметиламмония (CTAB) и аскорбиновая кислота. Некоторые из химических восстановителей, к сожалению, токсичны [33, 34, 36] и обычно пассивируются путем добавления на их поверхность стабилизаторов, таких как полиэтиленгликоль (PEG), гуммиарабик, полисахариды и биоактивные пептиды [37, 38].
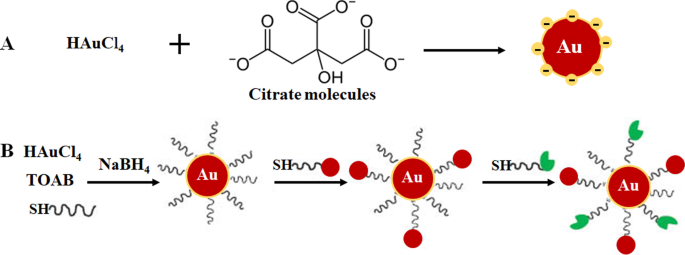
Состав AuNP через однофазную систему восстановлением цитрата ( A ) и двухфазное восстановление системы с последующей стабилизацией и функционализацией посредством реакции обмена лигандов, метод Бруста-Шиффрина ( B ). Воспроизведено с разрешения [36]. Авторское право 2013, De Gruyter. TOAB бромид тетрабутиламмония, SH тиолированные молекулы
Более экологичные подходы, такие как индуцированный микроволновым излучением процесс плазмы в жидкости (MWPLP) и зеленые нанотехнологии, были исследованы в синтезе AuNP, чтобы избежать использования токсичных химических восстановителей. MWPLP использует микроволны для зарождения металлических наночастиц и не требует никаких восстановителей, а энергия, необходимая для синтеза, очень мала [34]. Зеленая нанотехнология, с другой стороны, использует природные соединения, происходящие из растений и микроорганизмов, в качестве источника восстановителей при синтезе биогенных AuNPs [12, 33, 39,40,41]. Зеленые нанотехнологии считаются экологически чистыми и поэтому более подходящими для биомедицинских приложений. Опосредованный растениями синтез более экономичен, чем использование микроорганизмов. Более того, синтез можно выполнить всего за одну стадию, а наночастицы легче очистить. Кроме того, растения возобновляемы; различные части растений, такие как листья, стебли, кора, корни, цветы и плоды, можно собирать, не убивая растение, и использовать для синтеза. Экстракты, приготовленные из растительного материала, содержат фитохимические вещества, белки и ферменты, которые могут действовать как восстанавливающие, стабилизирующие и закрывающие агенты [10, 12, 24, 34, 35, 40, 42]. Эпигаллокатехин из зеленого чая [42] и мангиферин (MGF) из манго [12, 43] относятся к соединениям растительного происхождения, которые широко используются для синтеза AuNPs [34]. Более подробная информация об этих методах подробно рассмотрена в следующих ссылках [10, 24, 34, 35].
Биологическое применение AuNP
Роль и значение AuNP в медицинской науке, несомненно, становятся все более заметными, что подтверждается растущим числом исследований, демонстрирующих их многогранное применение в широком спектре биомедицинских областей. Биосовместимость AuNP объясняется долгой историей использования золота в лечении болезней человека, которая восходит к 2500–2600 годам до нашей эры. Китайцы и индийцы использовали золото для лечения мужской импотенции, эпилепсии, сифилиса, ревматических заболеваний и туберкулеза. Китай обнаружил эффект долголетия красного коллоидного золота, который до сих пор практикуется в Индии как часть аюрведической медицины для омоложения и оживления. Золото киновари (также известное как Макарадхваджа) используется в Индии для улучшения фертильности. В западных странах золото использовалось для лечения нервных расстройств и эпилепсии. О токсичности его использования не сообщалось ни в исследованиях in vitro, ни в исследованиях in vivo [8, 44, 45]. С тех пор оральные и инъекционные соединения золота продолжали использоваться для лечения артрита [9, 46], а также было показано, что они обладают противоопухолевым действием [8]. Аналогичные, а в некоторых случаях улучшенные эффекты были также зарегистрированы для AuNP, которые становятся многообещающими средствами для диагностики заболеваний [47, 48, 49] и терапии [3, 29, 50, 51].
AuNP имеют большую площадь поверхности, которую можно использовать в биомедицинских приложениях путем присоединения различных биомолекул для выполнения желаемой функции. Они могут включать нацеливающие группы, помогающие распознавать биомаркеры конкретных заболеваний, контрастирующие агенты для биовизуализации и терапевтические агенты для лечения заболеваний [24, 25]. Преимущество использования AuNP по сравнению с другими наноматериалами состоит в том, что они могут быть легко функционализированы с использованием различного химического состава, как показано на рис. 2 [4, 26]. AuNPs обладают высоким сродством к тиолированным молекулам, и связывание тиол-золото является наиболее часто используемым методом адсорбции молекул на поверхность NP [4]. Также используются химические процессы на основе аффинности, такие как связывание биотина и стрептавидина и карбодиимидное связывание. AuNP используются в трех основных областях биомедицины:доставка фармацевтических препаратов, диагностические и терапевтические цели [24, 35], и продемонстрировали огромный потенциал в этих областях, как обсуждается ниже.
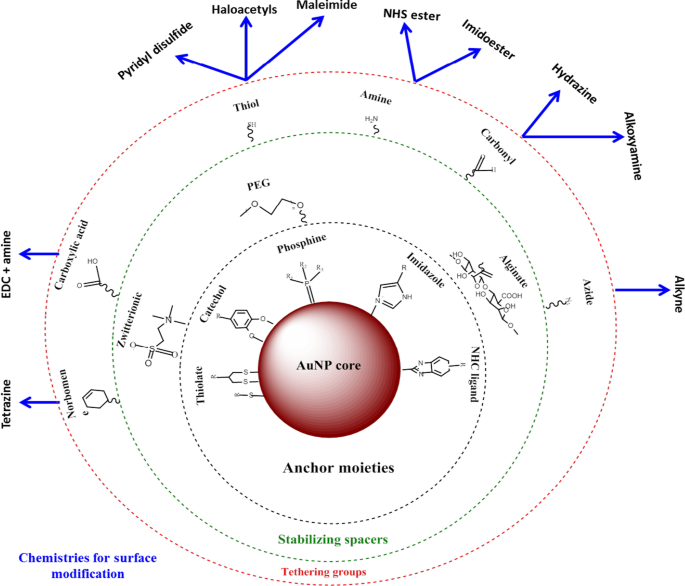
Синтез и функционализация AuNP. Биомолекулы с функциональными группами сначала адсорбируются на поверхности НЧ за счет сродства золото-тиол. Затем другие функциональные группы, такие как аминогруппа, могут быть использованы для связывания молекул с карбоксильными группами для присоединения нацеливающих или лекарственных групп. Адаптировано из [32]
AuNP как агенты по доставке лекарств
Чаще всего AuNP используются в качестве средств доставки лекарств [11, 18, 52], вакцин [53] и генной терапии [24, 32]. AuNP обладают свойствами, которые могут решить большинство проблем, связанных с традиционными методами лечения, таких как лекарственная устойчивость, низкое распределение лекарств, биодеградация и ранний клиренс лекарств [11]. AuNP могут значительно снизить дозировку лекарств, частоту лечения и способны транспортировать гидрофобные и нерастворимые лекарства. Они считаются биоинертными и могут маскировать свой груз от атаки иммунных клеток, защищать лекарства от протеолитической деградации при их перемещении по кровеносной системе и, таким образом, увеличивать время циркуляции лекарства. Эти факторы могут легко повысить эффективность лекарств, концентрируя и удерживая их в пораженных тканях, практически не влияя на нормальные ткани [25].
Использование AuNP в лечении рака широко изучалось [17, 37, 54], и с годами оно распространилось на другие заболевания, такие как ожирение [50, 55, 56] и угри [18]. Системы на основе наночастиц меньше, чем большинство клеточных компонентов, и могут пассивно проходить сквозь клеточные барьеры, используя эффект повышенной проницаемости и удерживания (EPR) на сосудистую сеть пораженных тканей [25]. EPR в патологическом состоянии характеризуется чрезмерным ангиогенезом и повышенной секрецией медиаторов проницаемости, которые могут усиливать поглощение AuNP пораженными тканями. Эти характеристики связаны только с патологическими состояниями, а не с нормальными тканями, что дает возможность избирательного воздействия на конъюгаты AuNP [25]. AuNP привлекательны в качестве носителей лекарств, поскольку они могут нести несколько молекул одновременно, что еще больше разнообразит их свойства. Это желательная черта в медицине, на которой основано большинство биологических приложений AuNP, поскольку AuNP могут быть адаптированы для конкретной биомедицинской функции. Это может помочь контролировать способ их взаимодействия с клеточными органеллами и, следовательно, дает надежду на будущую разработку эффективных методов диагностики и лечения различных заболеваний [4].
Диагностические системы на основе AuNP
Появление нанотехнологий повысило позицию в разработке систем обнаружения, которые являются быстрыми, надежными, чувствительными и высоко конкурентоспособными по сравнению с традиционными диагностическими тестами [48]. Наноматериалы обычно интегрируются в существующие биосенсорные платформы для обнаружения газов, ДНК и белковых маркеров, участвующих в развитии заболеваний [47]. Среди различных наноматериалов (которые включают металлические, полимерные, магнитные и полупроводниковые НЧ), используемых в диагностике, AuNP широко используются в биосенсорах, электрохимических датчиках и хромогенных анализах для обнаружения или определения наличия биомаркеров болезней [49]. Их локализованный SPR (LSPR), резонансный перенос энергии флуоресценции (FRET), поверхностное комбинационное рассеяние, проводимость, окислительно-восстановительная активность и эффект квантованной зарядки делают их идеальным инструментом для визуализации и обнаружения молекул-мишеней [10, 24]. Их электронные и оптические свойства, а также способность рассеивать видимый и ближний инфракрасный (NIR) свет совместимы и измеряются с помощью различных технологий, таких как микроскопические методы (электронное, конфокальное и темнопольное рассеяние света) [57], компьютерная томография (КТ). , PT-метод гетеродинной визуализации, УФ-видимая и рамановская спектроскопия [24, 35].
Разработка диагностических систем на основе AuNP включает модификацию поверхности AuNP, например, путем присоединения биомолекул, распознающих биомаркеры болезней [3, 24, 58]. Анализ бокового потока (LFA), вероятно, является самым известным примером диагностических инструментов, основанных на нанотехнологиях. LFA обычно используют AuNP размером около 30-40 нм, потому что более мелкие частицы имеют очень малое сечение экстинкции, тогда как более крупные частицы обычно нестабильны для использования в этих анализах [59]. Кроме того, включены другие молекулы / ферменты, которые могут запускать изменения в SPR, проводимости и окислительно-восстановительном потенциале AuNP. Эти индикаторы дают детектируемый сигнал после связывания аналитов с конъюгатами AuNP [24], отсутствие или присутствие сигнала затем будет отражать отсутствие или присутствие целевой молекулы или заболевания. Сигнал, генерируемый AuNP, является химически стабильным, длительным и постоянным при использовании в различных тестовых форматах:пробирке, полоске, in vitro и in vivo [24]. Следовательно, их применение значительно увеличило скорость и эффективность диагностических тестов.
Колориметрические анализы на основе AuNP
В колориметрических анализах AuNP производят визуальный сигнал (обычно изменение цвета), который можно обнаружить невооруженным глазом без использования современных инструментов. Как правило, коллоидный раствор AuNP имеет цвет от рубиново-красного до виноградного, который сильно зависит от расстояния между частицами [60, 61]. Связывание аналита с AuNPs, модифицированными элементами молекулярного био-распознавания (например, антителами, пептидами, аптамерами, ферментами и т. Д.), Вызывает отчетливый сдвиг LSPR, что, как следствие, приводит к изменению цвета с рубиново-красного на синий [60 , 62, 63]. Интенсивность цвета прямо пропорциональна концентрации аналита и используется для подтверждения наличия и состояния заболевания. Колориметрическая диагностика на основе AuNP успешно использовалась при обнаружении вируса гриппа A [64], вируса Зика [65], бактериофага T7 [66], Mycobacterium tuberculosis [67], а недавно - для выявления тяжелого острого респираторного синдрома - коронавируса-2 (SARS-CoV-2) [60, 68].
Пример колориметрического анализа на основе AuNP был продемонстрирован для обнаружения SARS-CoV-2 [60], вируса, вызывающего высокоинфекционное заболевание, вызванное вирусом короны 2019 (COVID-19) [60, 68]. С помощью этого анализа о присутствии вируса сообщали по простому изменению цвета; для диагностики не требовалось никаких инструментов. Текущие клинические диагностические тесты этого вируса используют либо анализ полимеразной цепной реакции в реальном времени с обратной транскриптазой (ОТ-ПЦР), который занимает 4-6 часов, тогда как системы быстрой диагностики (PoC) выявляют антитела, которые могут через несколько дней появиться в крови. Для сравнения, колориметрический анализ на основе AuNP был более надежным и быстрым, как показано на рис. 3. Инкубация AuNP, меченных антисмысловыми олигонуклеотидами (ASO), в присутствии образцов РНК SARS-CoV-2 приводила к образованию синего осадка внутри ~ 10 мин. В положительном тесте на SARS-CoV-2 связывание ASO с N-геном в нуклеокапсидном фосфопротеине вируса вызывало синий цвет, который определялся визуально. Тест был очень чувствительным и имел предел обнаружения РНК SARS-CoV-2 0,18 нг / мкл [60].
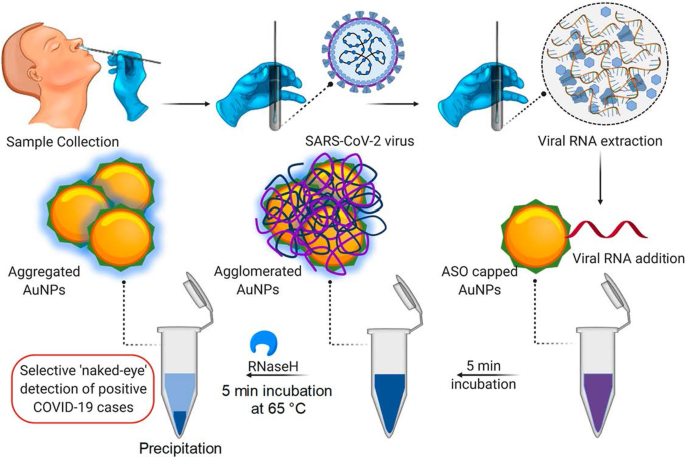
Колориметрическая диагностическая система на основе AuNP. Селективное обнаружение невооруженным глазом РНК SARS-CoV-2 с помощью AuNP с кэпом ASO. Воспроизведено с разрешения [60]. Авторские права 2020, ACS Nano
LFA на основе AuNP следуют тому же принципу, что и показанный на рис. 3; однако вместо изменения цвета раствора на тест-полоске формируется видимая линия, когда присутствует аналит. В присутствии аналита AuNP были захвачены на тестовой линии и образовали отчетливую красную линию, которую можно было визуализировать невооруженным глазом. Интенсивность линии определяется количеством адсорбированных AuNP [69]. Пример простого и быстрого LFA на основе AuNP показан на рис. 4 для обнаружения Pneumocystis jirovecii . ( П. jirovecii ) Антитела IgM в сыворотке крови человека. AuNP размером 40 нм конъюгировали с рекомбинантными синтетическими антигенами (RSA) P. jirovecii , либо основной поверхностный гликопротеин, либо кексин-подобная сериновая протеаза, которые использовались в качестве индикатора наличия или отсутствия P. jirovecii . В положительном тесте P. jirovecii IgM был захвачен конъюгатом AuNP-RSA на подушке конъюгата. Комплекс AuNP-RSA / IgM затем течет к аналитической мембране, где он связывает антитела против человеческого IgM (тестовая линия), а избыток перемещается к антителам против RSA (контрольная линия), в результате чего появляются две красные линии. Отрицательный тест будет иметь красный цвет только на контрольной линии [70]. В независимом исследовании использовались LFA на основе AuNP для выборочного обнаружения IgM к SARS-CoV-2, что подтверждается появлением красных линий как в тестовой, так и в контрольной линиях [68]. Цвет визуально определялся невооруженным глазом в течение 15 минут в двух системах, и для одного теста требовалось всего 10–20 мкл сыворотки [68, 70].
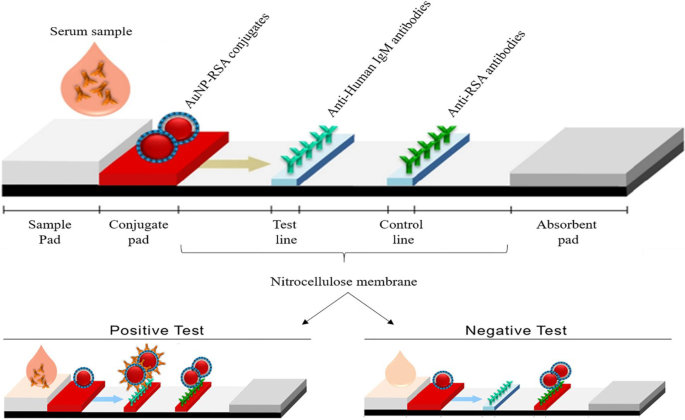
LFA на основе AuNP для обнаружения IgM P. jirovecii антитела. Наличие (положительный тест) или отсутствие (отрицательный контроль) P. jirovecii антитела можно было различить по красноватому цвету AuNP как в тестовой, так и в контрольной линиях или только в контрольной линии, соответственно. Воспроизведено с разрешения [70]. Авторское право 2019, Frontiers in Microbiology
Одним из первых примеров использования AuNP в качестве сигнального зонда на LFA было обнаружение клеток Рамоса; аптамер TE02 использовали в качестве зонда захвата, а аптамер TD05 - в качестве зонда обнаружения. Биосенсор аптамер-AuNP может визуально обнаружить минимум 4000 клеток Ramos без каких-либо инструментов и 800 клеток Ramos с помощью портативного стрип-ридера в течение 15 минут. Используя этот биосенсор для обнаружения сэндвич, анализ успешно обнаружил клетки Ramos в крови человека [71] и был использован в качестве доказательства концепции разработки быстрых, чувствительных и недорогих систем для качественного и количественного обнаружения циркулирующих раковых клеток. С тех пор различные LFA на основе AuNP были разработаны для диагностики множества инфекционных заболеваний, включая заболевания, вызываемые пневмоцистной пневмонией . [70], вирус Эбола [72], ВИЧ, вирус гепатита С и Mycobacterium tuberculosis [73] и совсем недавно вирус SARS-CoV-2 [68].
Системы визуализации на основе AuNP
AuNP интенсивно исследуются для применения в био-визуализации из-за их способности поглощать и рассеивать свет, соответствующий их резонансным длинам волн, до 10 5 раз больше, чем у обычных флуорофоров [74]. AuNP имеют более высокий атомный номер и электронную плотность (79 и 19,32 г / см 3 ) по сравнению с обычными препаратами на основе йода (53 и 4,9 г / см 3 ), тем самым оказавшись более эффективными контрастными веществами [24]. AuNP накапливаются на пораженных клетках или тканях и вызывают сильное ослабление рентгеновских лучей, делая целевой участок четко различимым и легко обнаруживаемым. AuNP прикреплены к химическим группам и молекулярным агентам биологического распознавания, которые могут избирательно нацеливаться на определенные антигены, чтобы индуцировать четкий и специфичный для мишени контраст для компьютерной томографии [75].
Нацеленная система молекулярной компьютерной томографии in vitro была достигнута с помощью AuNP, функционализированных аптамером РНК, который связывается с простатоспецифическим мембранным антигеном (PSMA). Конъюгат AuNP-PSMA-аптамер показал более чем четырехкратную интенсивность CT для PSMA-экспрессирующих клеток простаты (LNCaP) по сравнению с клетками предстательной железы PC-3, у которых отсутствует целевой рецептор [76]. Аналогичным образом, конъюгат AuNP-диатризойная кислота-аптамер AS1411 был локализован в клетках CL1-5 (аденокарцинома легкого человека) и в мышах, несущих опухоль CL1-5. Аптамер AS1411 нацелен на рецептор нуклеолина (NCL), который экспрессируется клетками CL1-5 на поверхности клетки, в то время как диатризойная кислота представляет собой контрастное вещество на основе йода. Конъюгат AuNP – диатризойная кислота – аптамер AS1411 имел линейную кривую ослабления с наклоном 0,027 мМ Au Hounsfield (HU −1 ), что указывает на накопление AuNP в месте опухоли [77]. AuNP демонстрируют более длительное время удерживания в сосудах, что увеличивает время их циркуляции в крови [77,78,79] и улучшает сигнал CT диатризойной кислоты [77].
На Фигуре 5 показана in vivo КТ-визуализация сосудов коронарных артерий с использованием AuNP, которые были конъюгированы с связывающим коллаген адгезионным белком 35 (CNA35) для нацеливания на коллаген I при инфаркте миокарда у грызунов. Сигнал AuNP все еще обнаруживался в крови через 6 ч после внутривенного (в / в) введения, что было значительно выше, чем период полувыведения (5–10 мин) препаратов на основе йода [79]. Эти эффекты были воспроизведены с использованием синтезированных зеленым маннан-кэпированных AuNP, которые показали опосредованное рецептором захват и нетоксичность в клетках, экспрессирующих маннозу (DC 2.4 и RAW 264.7). AuNP с кэпом маннана избирательно нацелены на подколенные лимфатические узлы in vivo после инъекции в заднюю ногу мышей [38]. КТ на основе AuNP может предоставить важную информацию для диагностики различных заболеваний, не ограничиваясь коронарной артерией и раком [76, 77, 79, 80, 81]. Использование AuNP в качестве контрастных агентов показало потенциал в других системах визуализации, таких как фотоакустическая, ядерная, ультразвуковая и магнитно-резонансная томография. Эти системы подробно рассматриваются в других источниках [82, 83].
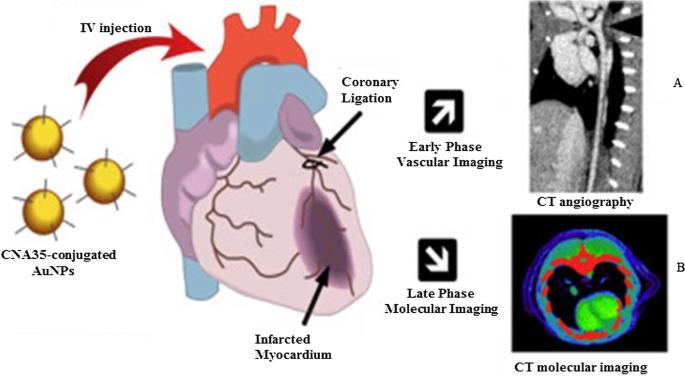
КТ in vivo с использованием AuNP в качестве контрастных агентов для КТ. AuNP с маннановыми капиллярами и их компьютерная томография лимфатических узлов ( A ) и CNA35-конъюгированные AuNPs КТ бремени миокарда ( B ). Воспроизведено с разрешения [79]. Авторские права 2018, Elsevier
AuNP в флуоресцентных системах обнаружения
AuNP используются в системах обнаружения на основе флуоресценции в качестве флуоресцентных агентов или гасителей флуоресценции. При размерах ≤ 5 нм AuNP проявляют свойства квантовых точек (КТ) и могут использоваться вместо них. Au 55 (PPh 3 ) 12 Cl 6 нанокластеры, введенные в 1981 г., вероятно, наиболее интенсивно изучаются из-за их квантово-размерного поведения [7]. С тех пор различные AuNP квантового размера (AuNPsQ), такие как Au 25 (SR) 18 , Au 38 (SR) 24 и Au 144 (SR) 60 [84] изучались в основном в области электрохимического зондирования, поскольку они являются отличными электронными проводниками и окислительно-восстановительными медиаторами [85].
Пленочные электроды AuNPsQ были использованы при изготовлении сверхчувствительного электрохимического иммуносенсора для обнаружения простатоспецифического антигена (PSA). Иммуносенсор имел чувствительность 31,5 мкА мл / нг и предел обнаружения 0,5 пг / мл для ПСА в 10 мкл неразбавленной сыворотки крови человека. Иммуноанализ показал результаты в восемь раз лучше, чем ранее сообщенный лесной иммуносенсор на углеродных нанотрубках, содержащий несколько компонентов, при концентрации биомаркера, которая была ниже, чем уровни, связанные с наличием рака. Таким образом, его можно использовать для измерения тестового биомаркера как в нормальном, так и в болезненном состоянии. Характеристики иммуносенсора были сопоставимы с эталонным методом ELISA [86]. AuNPsQ также был включен в пористую структуру CaCO 3 сферы с образованием флуоресцентного CaCO 3 / Гибрид AuNPsQ для обнаружения нейрон-специфической енолазы, диагностического и прогностического биомаркера черепно-мозговой травмы и рака легких. Предел обнаружения датчика составлял 2,0 пг / мл -1 . [87]. До сих пор сообщалось о нескольких системах флуоресцентного обнаружения на основе AuNP для обнаружения аналитов, связанных с гепатитом B [73, 88], гриппом A [89], раком [90] и повреждением сердца [91].
AuNP также являются отличными тушителями на основе FRET [92]. Их уникальные оптические свойства (стабильная интенсивность сигнала и сопротивление фотообесцвечиванию), размер и способность к модификации сделали их привлекательными зондами в платформах для определения флуоресценции [93, 94]. Более крупные AuNP (≥ 10–100 нм) имеют низкие квантовые выходы, которые не подходят для прямого флуоресцентного зондирования; однако их способность гасить флуоресцентные красители в состоянии с относительно высокой энергией возбуждения сделала их эффективными гасителями фотолюминесценции [94]. В принципе, флуоресцентные нанозонды состоят из донорного флуорофора (красителя или квантовых точек) и акцепторных AuNP, и когда они находятся в непосредственной близости, флуоресценция выбранного флуорофора подавляется AuNP [94, 95]. В отсутствие мишени, на что указывает отсутствие флуоресцентного сигнала, зонд нуклеиновой кислоты гибридизуется и образует петлевую структуру, которая сближает флуорофор и гаситель на его противоположных концах; в то время как связывание аналита с зондом нуклеиновой кислоты вытесняет флуорофор из AuNP, что приводит к флуоресцентному сигналу [24, 94, 96]. Используя преимущества вышеупомянутых свойств, AuNP были включены в молекулярные маяки для in vitro (золотые наносферы, AuNS) и in vivo (золотые наностержни, AuNR) обнаружения экспрессии матриптазы на опухолевых клетках. Два молекулярных маяка состояли из сайта расщепления матриптазы в качестве линкера между AuNP и флуорофорами. Молекулярный маяк AuNS был сконструирован с использованием изотиоцианата флуоресцеина (FITC), а молекулярный маяк AuNR имел флуоресцентный краситель NIR (меркаптопропионовая кислота, MPA). In the absence of the target, the AuNSs and AuNRs, respectively, blocked the FITC and MPA fluorescence. Cleavage of either FITC or MPA from the AuNP–molecular beacons in the presence of matriptase exhibited a quantifiable fluorescence signal. The fluorescent signal of the MPA–AuNR–beacon in the nude mice bearing HT-29 tumors lasted for 14 h in the tumor site, while the signal gradually disappeared from the non-tumor site over time [97].
The AuNPs were reported to have comparable or higher fluorescence quenching efficiency than organic quenchers such as 4-((4′-(dimethyl-amino)phenyl)azo)benzoic acid (DABCYL) [94, 98] and Black Hole Quencher-2 [99]. The fluorescence quenching efficiency of 1.4 nm AuNPs was compatible with the four commonly used organic fluorophores (FITC, rhodamine, texas red and Cy5). The fluorescence quenching efficiency of the AuNPs was similar to that of DABCYL, and unlike DABCYL, the AuNPs showed consistency in both low and high salt buffers [98]. In a competitive hybridization assay, 10 nm AuNPs showed superior (> 80%) fluorescence quenching efficiency for Cy3 dye than the commercial Black Hole Quencher-2 (~ 50%). The assay had a limit of detection of 3.8 pM and a detection range coverage from 3.8 pM to 10 nM for miRNA-205 in human serum, and it was able to discriminate between miRNAs with variations in their nucleotide sequence [99]. The competitive sensor arrays were not only sensitive [96, 99] but were able to differentiate between normal and diseased cells, as well as benign and metastatic cancers [96].
AuNP-Based Bio-barcoding Assay
AuNP-based bio-barcoding assay (BCA) technology has become one of the highly specific and ultrasensitive methods for detection of target proteins and nucleic acids up to 5 orders of magnitude than the conventional assays [100]. The assay relies on magnetic microparticle probes, which are functionalized with antibodies that bind to a specific target, and AuNP probes encoded with DNA that recognizes the specific protein target and antibodies. Upon interaction with the target DNA, a sandwich complex between the magnetic microparticle and AuNPs probes is formed. The sandwich is then separated by the magnet followed by thermal dehybridization to release the free bar-code DNA, enabling detection and quantification of the target [101, 102].
The AuNP-based BCA assay was able to detect HIV-1 p24 antigen at levels that was 100–150-fold higher than the conventional ELISA [103]. The detection limit of PSA using these systems was 330 fg/mL [104]. The versatility of AuNPs for the development of a BCA-based platform was further demonstrated by measuring the concentration of amyloid-beta-derived diffusible ligands (ADDLs), a potential Alzheimer's disease (AD) marker found in the cerebrospinal fluid (CSF). ADDL concentrations were consistently higher in the CSF taken from the subjects diagnosed with AD than in non-demented age-matched controls [105]. These results indicate that the universal labeling technology can be improved through the use of AuNPs to provide a rapid and sensitive testing platform for laboratory research and clinical diagnosis.
AuNP-Based Therapies
Metal-based drugs are not new to medicine; in fact, they are inspired by the existing metallic drugs used in clinical treatment of various diseases [9, 106,107,108,109]. The widely studied and clinically used metal-based drugs were derived from platinum (e.g., cisplatin, carboplatin, tetraplatin for treatment of advanced cancers), bismuth (for the treatment of infectious and gastrointestinal diseases), gold (for the treatment of arthritis) and gallium (for the treatment of cancer-related hypercalcemia) [108, 109]. The approval of cisplatin in 1978 by the FDA for the clinical treatment of cancer [107] further inspired research on other metals (such as palladium, ruthenium, rhodium) [32, 106, 110].
Owing to the bioactivities, which included anti-rheumatic, antibacterial and anticancer effects, and the biocompatibility of bulk gold [8, 9, 46, 111], AuNPs are extensively investigated for the treatment of several diseases. AuNPs displayed unique and novel properties that are superior to its bulk counterpart. AuNPs are highly stable and have a distinct SPR, which guides their application in medicine [112], as drug delivery and therapeutic agents. AuNPs have a lot of advantages over the conventional therapy; they have a longer shelf-life and can circulate long enough in the system to reach their targets [25] with [11, 49, 113] or without targeting molecules [14, 15, 24, 25, 114]. AuNPs can provide localized and selective therapeutic effects; some of the areas in which AuNPs were used in therapy are described below.
Therapeutic Effects of Untargeted AuNPs
The as-synthesized (i.e., unmodified or uncapped) AuNPs have been shown to have diverse therapeutic effects against a number of infectious [115, 116], metabolic and chronic diseases [3, 29, 50, 51]. Their antioxidant, anticancer, anti-angiogenic [3, 32], anti-inflammatory [3, 51] and weight loss [29, 50, 112] effects are beneficial for diseases such as cancer, rheumatoid arthritis, macular degeneration and obesity [5, 25, 113, 117]. The above-mentioned diseases are characterized by a leaky vasculature and highly vascularized blood vessels [5, 113], which provides the NPs an easy passage into the diseased tissues and increase the susceptibility of cells to their effects. Through the EPR effect, uncapped AuNPs can passively accumulate in the vasculature of diseased cells or tissues. Hence, AuNPs have been specifically designed to have anti-angiogenic effects in diseases where angiogenesis (the growth and extension of blood vessels from pre-existing blood vessels) spins out of control like cancer, rheumatoid arthritis, macular degeneration and obesity [5, 25, 113, 117]. Targeting and destroying the defective blood vessels prevent oxygen and nutrients from reaching the diseased cells, which results in their death. The pores in the blood vessels at the diseased site (especially in cancer and obesity) are 200–400 nm and can allow materials in this size range to pass from the vasculature into the diseased tissues and cells [14, 15, 25, 114].
The cellular uptake, localization, biodistribution, circulation and pharmacokinetics of the uncapped AuNPs rely strongly on size and shape [49]. Although these effects are applicable to all AuNPs, the biological effects of citrate-capped AuNPs (cAuNPs) are extensively studied and reviewed. Spherical cAuNPs demonstrated selective in vitro anticancer activity that was size and concentration dependent on murine and human cell lines [3, 51]. Different sizes (10, 20 and 30 nm) of cAuNPs showed differential effects in human cervical carcinoma (HeLa), murine fibroblasts (NIH3T3) and murine melanoma (B16F10) cells. The 20 and 30 nm cAuNPs showed a significant cell death in HeLa cells starting at the lowest concentration of 2.2 µg/mL, while the 10-nm NPs was toxic at concentrations ≥ 8.75 µg/mL. The activity of these NPs was negligible in the noncancerous NIH3T3 cells, especially the 10 and 20 nm. The 20 nm reduced viability by ≤ 5% at the highest concentration (35 µg/mL), and ~ 20% for the 10 and 30 nm. The IC50 values for 10, 20 and 30 nm cAuNPs in the Hela cells were 35, 2.2 and 4.4 μg/mL, respectively, while the IC50 values for noncancerous cells were higher than 35 µg/mL [3]. Using a concentration range of 0.002–2 nM, 13 nm cAuNPs induced apoptosis in rabbit articular chondrocytes and no effects were observed for 3 and 45 nm cAuNPs under the same conditions. The 13 nm cAuNPs induced mitochondrial damage and increased reactive oxygen species (ROS); these actions could not be blocked by pre-treatment with a ROS scavenger, the N-acetyl cysteine [51]. Size-dependent effects were also observed in vivo after injecting cAuNPs of various sizes (3, 5, 8, 12, 17, 37, 50 and 100 nm) into mice (8 mg/kg/week) for 4 weeks. The 8, 17, 12 and 37 nm were lethal to the mice and resulted in tissue damage and death after 14 days of treatment; the other sizes were not toxic and the mice survived the experimentation period. On the contrary, the same-size AuNPs at a concentrations up to 0.4 mM were not toxic to HeLa cells after 24 h exposure [118].
The cAuNPs can interact and accumulate nonspecifically within various tissues and organs in the body, especially in the reticuloendothelial system (RES) organs (blood, liver, spleen, lungs) [55, 119]. This was evident in high-fat (HF) diet-induced obese Wistar rats [55] and Sprague–Dawley rats [119] following acute (1 dose for 24 h) [55] and chronic (1 dose; 0.9, 9 and 90 µg/week over 7 week period) [119] exposure to 14 nm cAuNPs, respectively. Majority of the i.v injected cAuNPs were detected in the liver, spleen, pancreas, lungs, kidneys [55, 119] including the skeleton and carcass of the rats [119]. Chen et al. observed that after intraperitoneal (i.p) injection of a single dose (7.85 µg/g bodyweight) of 21 nm cAuNPs in lean C57BL/6 mice, they accumulated in the abdominal fat tissues and liver after 24–72 h [29], as well as the spleen, kidney, brain and heart in the HF-induced obese mice that were injected with the same dose daily for 9 weeks [50]. The cAuNPs reduced the abdominal WATs (retroperitoneal and mesenteric) mass and blood glucose levels 72 h post-injection [29]. In the diet-induced obese mice, the 21 nm cAuNPs demonstrated anti-inflammatory and anti-obesity effects [50]. They also improved glucose tolerance, enhanced the expression of inflammatory and metabolic markers in the retroperitoneal WATs and liver [50]. Both the 14 and 21 nm cAuNPs showed no sign of toxicity or changes in the markers associated with kidney and liver damage [29, 55, 119].
Similar findings were reported for plant-mediated AuNPs, without targeting molecules they can access, ablate tumors [40, 120] and obese WATs [121] in rodents. Differential uptake, distribution and activity of biogenic AuNPs also vary depending on the size and shape of the NPs. While certain sizes can pass through the vascular network and be retained at the site of the disease; others can be easily filtered out of the system through the RES organs and the mononuclear phagocytic system as shown in Fig. 6 [15, 114]. NPs can be removed by tissue-resident macrophages (TRMs) before they reach the disease cells. Those that escape the TRMs and do not reach the disease site, especially smaller NPs (≤ 5 nm), are excreted through glomerular filtration in the kidney [25, 114]. Pre-treatment with clodronate liposomes depleted the TRMs in the liver and spleen before exposure to 50, 100 and 200 nm AuNPs. This reduced uptake of the AuNPs by the liver, increased their half-life in the blood as well as their accumulation at the tumor site [122]. However, TRMs are not the only obstacle that the AuNPs that rely on EPR effect for uptake must overcome. EPR effect alone can only ascertain ≤ 1% AuNP uptake [15, 114], and depletion of the TRMs prior to treatment resulted in just ≤ 2% of NPs reaching the target [122]. The success of non-targeted AuNPs depends on their ability to reach and accumulate in the diseased tissues, of which passive targeting through the EPR effect might not be efficient. The NPs also need to circulate longer, escape early clearance, and most importantly show reduced bystander effects [25, 123]. These qualities can increase bioavailability and ensure selectivity and efficacy of the AuNPs. These can further be improved by changing the surface chemistry of the AuNPs as discussed below [15, 124].
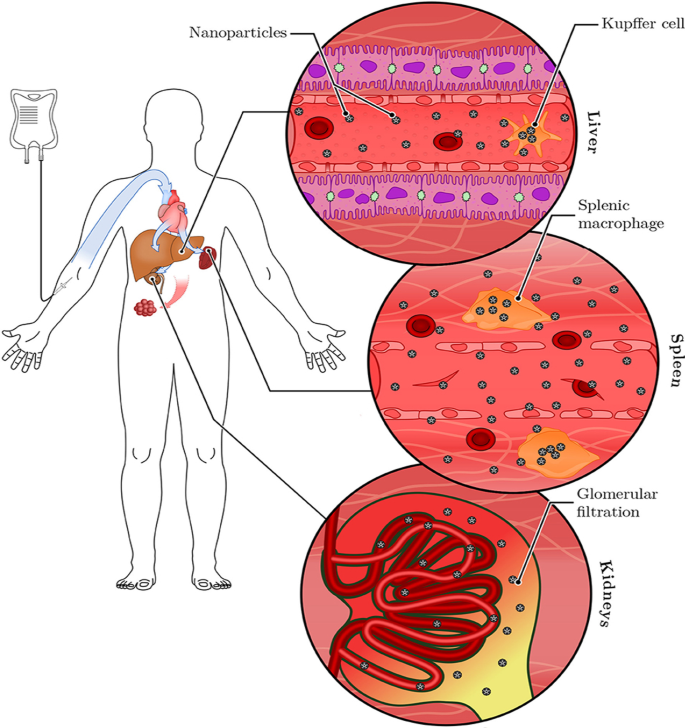
RES-based clearance of systemic administered AuNPs depends on their size. Large AuNPs accumulate in the liver, while smaller AuNPs are likely to end up in the spleen or be excreted in the urine via glomerular filtration. The AuNPs that escape the TRMs could accumulate in the diseased tissues. Reproduced with permission [114]. Copyright 2019, Frontiers in Bioengineering and Biotechnology
Therapeutic Effects of Surface-Functionalized AuNPs
The common strategy in AuNP-based therapeutics involves modifying the AuNP surface with therapeutic agents [3, 124,125,126]. The therapeutic agents can be drugs already used for the treatment of a particular disease or biomolecules with known inhibitory effects on cell signaling. In some instances, the therapeutic AuNPs have also been designed to have molecules that facilitate active targeting of the AuNPs toward specific cells and tissues. The molecules can easily adsorb on the AuNP surface by thiolation, chemical modification using chemistries such as 1-ethyl-3-(3-dimethylaminopropyl) carbodiimide (EDC), streptavidin/biotin binding [3, 124,125,126,127] and ionic interactions based on opposite charges between the NP surface and the biomolecules [124,125,126]. Functionalization of the AuNP surface influences their physicochemical properties and can affect their safety, biocompatibility and mobility. To ensure that the cargo carried by the AuNPs is delivered to the intended site, consideration should thus be given to both the physical and chemical properties of the AuNPs [124,125,126]. It is especially the size, shape, charge and the capping agents of the AuNPs that play an important role in the functionality of the AuNP conjugates [124] and can completely alter the pharmacokinetics of the AuNP-based therapeutics.
Functionalization allows for the development of customized nanosystems to reduce undesirable bystander effects often associated with traditional medicine. Functionalization of AuNPs can also prevent nonspecific adsorption of proteins onto the AuNP surface which can result in the formation a protein corona, resulting in the early clearance of the AuNPs through opsonization by the phagocytic cells [49, 123]. The surface charge of NPs can have a major influence on the behavior of NPs within biological environments. AuNPs with a neutral surface charge are unreactive and have a higher rate of escaping opsonization than charged AuNPs. Hydrophilic NPs will also behave differently to those with hydrophobic surfaces [49, 123]. PEG is one of the polymers most often used to mask AuNPs from phagocytic cells and has been shown to stabilize and enhance the biocompatibility of the AuNPs in numerous in vivo studies [49, 55, 123]. Pegylation improved the biocompatibility of 8.2 nm AuNPs by preventing neutrally and negatively charged AuNPs to bind to cell membranes or localize to any cellular components in African green monkey kidney (COS-1) cells [49]. And when the pegylated AuNPs were functionalized with a polyarginine cell penetrating moiety, the AuNPs were visualized on the cell membrane and inside the COS-1 cells [49]. Cell-penetrating peptides such as nuclear localization signal from SV40 virus, Tat from HIV and polyarginine peptides have been explored in translocation of AuNPs inside all cell type, normal or diseased. However, high specificity is required for clinical applications and can be achieved by taking advantage of the physiological differences between malignant and normal cells. This has been achieved by functionalizing the AuNPs with targeting molecules that recognize cell-specific receptors that are exclusively or overexpressed on the surface of target cells. This way, the AuNPs can be directed and delivered only to cells that express the target receptor. Therefore, conjugation of targeting moieties to the AuNPs (active targeting) will provide more selectivity, reduced bystander toxicity and enhanced efficacy since the AuNPs will be confined only to malignant tissues that express the target receptors [49, 55, 57, 113, 126, 127].
A good example to demonstrate the versatility of AuNPs is shown in Fig. 7, where four different molecules were conjugated onto the AuNPs to target two independent markers and mechanisms [11]. The multifunctional AuNPs were used for the treatment of leukemia (K562DR) cells that are resistant to doxorubicin (Dox). The 40 nm AuNPs were modified with two targeting moieties (folate and AS1411 aptamer) and two therapeutic agents (Dox and anti-miRNA molecules/anti-221). Folate molecule and AS1411 aptamer, respectively, recognize the folate and NCL receptors that are overexpressed on the cell surface and through receptor-mediated endocytosis will traffic the AuNP-conjugate into the cells. The AS1411 aptamer had dual functions, by also targeting the NCL receptor that is expressed inside the cells. After the AuNP-conjugate has been shuttled into the cells, the cargo (AS1411 aptamer, anti-221 and Dox) is off-loaded which independently act on three mechanisms that will synergistically bring about the demise of the cells. AS1411 aptamer together with anti-221 prevented leukemogenesis by suppressing the endogenous NCL and miR-221 function in the NCL/miR-221 pathway, thereby sensitizing the cells to the effects of Dox [11].
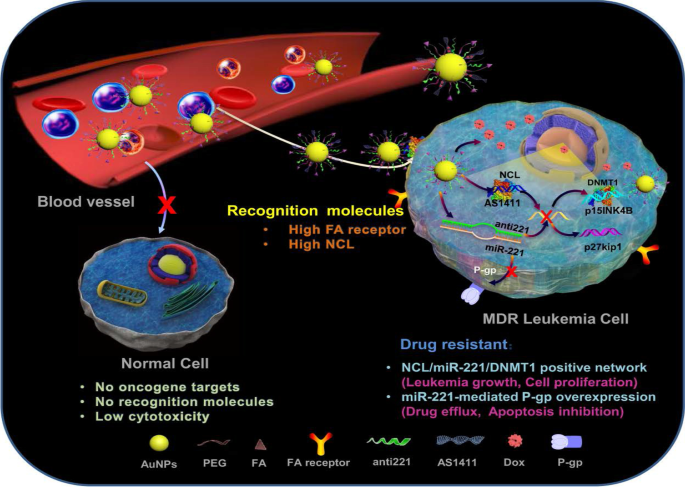
Multifunctional AuNPs in the treatment of multidrug-resistant (MDR) leukemia cells by increasing the sensitivity of the cells to Dox. Reproduced with permission [11]. Copyright 2019. Springer Nature. Folate (FA) receptor
Interestingly, similar dual targeting and treatment effects were achieved with green synthesized AuNPs without any additional molecules. With natural products acting as reducing agents, the biogenic AuNPs might also be more biocompatible than the chemically synthesized NPs [12, 40, 41, 43, 120]. MGF-AuNPs selectively targeted the laminin receptors in prostate (PC-3) and triple-negative breast cancer (MDA-MB-231) cells, and their xenografts in severe combined immunodeficiency (SCID) mice bearing these tumors [12, 40, 120]. In the normal SCID mice, the majority (85% at 30 min increasing to 95% after 24 h) of the i.v-injected MGF-AuNPs accumulated in the liver. Less than 10% were detected in the blood (2.7%), spleen (5%), lungs (0.6%), stomach, intestines and kidneys. When intra-tumorally injected in SCID mice-bearing prostate tumors, only 11% of the MGF-AuNPs were detected in the liver 24 h post-injection, while ~ 80% was in the tumor. Negligible amounts were found in the stomach, carcass and the small intestines. Some of the AuNPs were excreted through the renal and hepatic pathways in the urine and feces after 24 h [40, 120]. Nano Swarna Bhasma, a mixture consisting of AuNPs synthesized from mango peel extracts and phytochemicals from mango, turmeric, gooseberry and gum arabic, showed reduced toxicity toward normal endothelial cells after 48 h compared to the MDA-MB-231 cells [12].
Several studies have demonstrated that AuNPs have potential for clinical application. In combination with conventional drugs, it can be used to sensitize diseased cells to the drug effects [12, 128] and also prevent or reduce drug-related bystander effects [12]. AuNPs improved the pharmacokinetics of chemotherapeutic drugs, such as Dox [43, 129] and 5-fluorouracil (5-FU) [128]. Great improvements were mostly seen in the permeability and retention of drugs in the diseased cells, resulting in enhanced efficacy [130]. Dox-loaded AuNPs, which were non-toxic toward normal mouse fibroblast (L929) cells, also demonstrated selective toxicity toward fibrosarcoma tumors in mice [129]. 5-FU conjugated to the cAuNPs had better activity than 5-FU on its own in colorectal cancer cells [128]. AuNP co-treatment with chemotherapeutic drugs was highly efficient in improving the efficacy of chemotherapeutic drugs [12, 43, 128, 129, 131]. Orally ingested Nano Swarna Bhasma in combination with Dox and Cyclophosphamide reduced tumor volumes in SCID mice-bearing breast tumor cells and also showed acceptable safety profile and reduced bystander effects of the chemotherapeutic drugs in stage IIIA/B metastatic breast cancer patients [12]. Active targeting alone can ensure that the AuNPs are directly delivered into the desired targets, achieving a balance between efficacy and toxicity while minimizing damage to healthy tissues [14, 15, 49]. Controlled drug release is also among the many advantages offered by the AuNP-based systems and is crucial as it allows for localized and selective toxicity [49]. The AuNPs can be designed in such a way that their conjugates respond to internal (glutathione displacement, enzyme cleavable linkers, pH) or external (light, heat) stimuli to function [24, 25, 34, 128].
AuNPs as Transfection Agents in Gene Therapy
The use of AuNPs in gene therapy has shown promising outcomes by facilitating the delivery of genetic material to cells to silence or enhance expression of specific genes [24, 32, 132]. Thus, AuNPs can be used as transfection reagents in gene therapy for the treatment of cancer and other genetic disorders. AuNP conjugates have demonstrated higher transfection efficiency than experimental viral and non-viral gene-delivery vectors including polycationic reagents that has been approved for clinical use [24].
AuNPs are highly conductive and well suited for use as microelectrodes during electroporation for intracellular delivery of biomolecules for disease treatment. AuNPs significantly enhanced the performance of electroporation systems and have been used successfully for the delivery of DNA into hard-to-transfect cells such as the K562 cells [133]. To prevent cell loss which is often associated with electroporation, targeting moieties can be conjugated to the AuNPs to facilitate cellular uptake of AuNP conjugates through receptor-mediated mechanisms [133]. The use of AuNPs to transfect cells with oligonucleotide molecules also has the added advantage of increasing the half-life of these biomolecules and their efficacy [24, 32].
Untargeted AuNP conjugates are passively transported into cells and rely on the surface charge and AuNP shape for efficient transfection [24, 36, 134, 135]. The charge of the biomolecules that are conjugated onto AuNP surface plays a crucial role in their transfection efficiency; for instance, AuNPs functionalized with cationic molecules produce higher transfection efficiency than AuNPs functionalized with anionic molecules. Positively charged amino acids (lysine) can be attached on the NP surface to increase the rate of transfection. AuNSs [24] and AuNRs [36, 134, 135] are commonly used for transfections, and relative to the conventional transfection reagents (X-tremeGENE and siPORT), they inhibited the expression of target gene by > 70% in vitro [134] and in vivo [135]. In these studies, transfection efficiency was quantified based on target expression using RT-PCR and immunostaining [134, 135]. As transfection reagents, AuNPs provide long-lasting effects, localized gene delivery and higher efficacy [36, 134, 135]. Other types of nanomaterials (e.g., polymeric, liposomes, ceramic and carbon nanotubes) had received more attention for use in gene therapy than AuNPs. Six clinical trials using either polymeric or lipid-based nanomaterials for delivery of siRNA in solid tumors have been completed [36, 134, 136]. All of which suffer from low loading efficiency, low stability, and insufficient payload release [36, 136]. On the other hand, transfection systems based on AuNPs make use of easy chemistry that ensures efficient loading capacity and formation of stable complexes [36, 135]. Their safety can be controlled by manipulating their shape, size distribution and surface composition [36].
Antimicrobial Effects of AuNPs
MDR microbes are a major health concern and a leading cause of mortality, worldwide [21, 137,138,139,140,141]. These microorganisms have become resistant to conventional antimicrobial agents, due to over-prescription and misuse of these drugs [142]. No new antibiotics have been produced in over 40 years, mainly because the big pharmaceutical companies have retreated from their antibiotic research programs due to the lack of incentives [143]. As such, new and effective antimicrobial agents are urgently required to combat what could be the next pandemic, the antimicrobial resistance, and avoid surge in drug-resistant infections.
AuNPs are among the new generation of antimicrobial agents under review. They have shown broad antimicrobial (bactericidal, fungicidal and virucidal) effects against a number of pathogenic and MDR microorganisms and thus have potential to overcome microbial drug resistance [21, 142, 144]. Their antimicrobial effects are dependent on their physicochemical properties, especially their size, surface composition, charge and shape [21, 144]. Due to their small size, AuNPs can easily pass through the bacterial cell membrane, disrupt their physiological functions and induce cell death [35]. The exact antimicrobial mechanisms of AuNPs are not yet fully elucidated; despite this, some of the reported modes of actions that results from the interaction of various nanostructured materials (NSMs) with the bacterial cells are illustrated in Fig. 8. The highlighted mechanisms are also implicated in antimicrobial activity of AuNPs, they include induction of microbial death through membrane damage, generation of ROS and oxidative stress, organelle dysfunction, and alteration of gene expression and cell signaling [141].
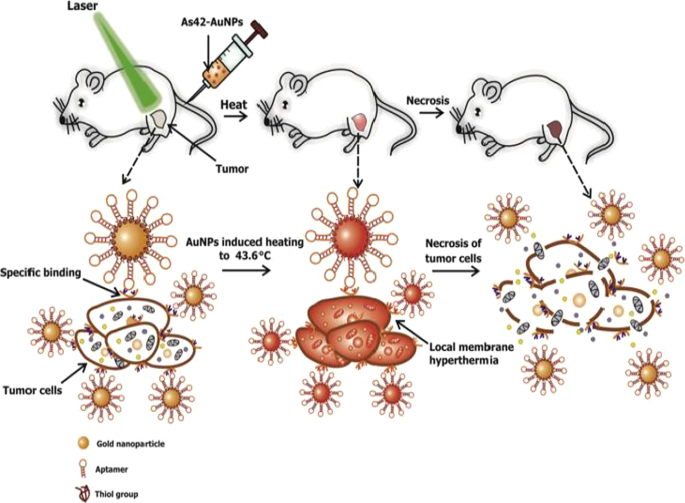
Antimicrobial mode of actions of the NSMs. Various NSMs can induce cell death by altering various biological functions, X represents alteration of cell signaling by de-phosphorylation of tyrosine residues in proteins as one of the mechanisms. Reproduced with permission [141]. Copyright 2018, Frontiers in Microbiology
AuNPs have multiple roles to play toward the development of antimicrobial agents, aside from being antimicrobial agents by themselves; they can serve as drug sensitizers and drug delivery vehicles [35, 58, 132, 145]. These features are applicable to both the chemical and green synthesized AuNPs, which have been reported to have antimicrobial effects against a number of human [21, 145,146,147] and waterborne [148] pathogenic strains. Generally, the test bacteria had shown low susceptibility toward the chemically synthesized AuNPs, i.e., the cAuNPs [21, 146, 147] and the NaBH4 -reduced AuNPs [149]. This was due to the repulsive forces between the negative charges on the AuNP surfaces and bacterial cells, thus preventing the interaction between AuNPs and the bacteria [21]. The activity of chemically synthesized AuNPs is based on their size, shape, concentration and exposure time. As an example, one study reported that NaBH4 -reduced AuNPs had no activity against Staphylococcus aureus (S. aureus ) and Escherichia coli (E. coli ) at 500 µg/mL for the duration of 6 h [149]. In contrast, another study showed a significant dose (1.35, 2.03 and 2.7 μg/mL) and size (6–34 nm vs 20–40 nm) dependent antibacterial effects of the NaBH4 -reduced AuNPs on Klebsiella pneumonia , E. coli , S. aureus and Bacillus subtilis [145].
The AuNPs are either used alone or in combination with other antimicrobial agents to treat microbial infections [35, 58, 132, 145]. When used in combination with other antimicrobial agents, the AuNP conjugates resulted in synergistic antimicrobial effects that surpassed the individual effects of the AuNPs and drugs [21, 35, 58, 132, 150]. These drugs were conjugated onto the AuNPs by either chemical methods [4, 151] or the drugs were used as reducing and capping agents [21, 149]. By so doing, the AuNPs improved drug delivery, uptake, sensitivity and efficacy. Some of the FDA-approved antibiotics and non-antibiotic drugs that were loaded onto the AuNPs are shown in Table 1 [4, 21, 149, 152]. Ciprofloxacin [152], cefaclor [149], lincomycin [4], kanamycin [21], vancomycin, ampicillin [151] and rifampicin [32] are among the antibiotics loaded on the AuNPs and demonstrated the versatility of AuNPs. These strategies were successful with various sizes and shapes of AuNPs, including gold silica nanoshells [152], AuNP-assembled rosette nanotubes [151] and AuNPs encapsulated in multi-block copolymers [153]. For instance, cefaclor-reduced AuNSs inhibited the growth of S. aureus and E. coli within 2–6 h depending on the concentration (10–50 µg/mL), while complete bacterial growth inhibition by the drug alone was only observed at 50 µg/mL after 6 h. The minimum inhibitory concentration (MIC) of the treatments was 10 µg/mL and 50 µg/mL for cefaclor-AuNPs and cefaclor, respectively [149].
AuNPs have presented properties that make them ideal candidates as alternative antimicrobial agents; the most important being their broad antimicrobial activity [21, 35, 58, 132, 150]. Owing to their biocompatibility and easily modifiable surface, microorganisms are less prone to developing resistance toward AuNPs [21]. For example, the kanamycin (Kan)-resistant bacteria (S . bovis , S . epidermidis , E . aerogenes , P . aeruginosa and Y . pestis ) showed increased susceptibility toward Kan-reduced AuNPs. The MIC values for Kan-AuNPs on the test bacteria were significantly reduced to < 10 µg/mL when compared to the MIC values for Kan alone at 50–512 µg/mL. This shows that AuNPs can restore the potency of antibiotics toward the drug-resistant strains by facilitating the uptake and delivery of the antimicrobial agents [21]. AuNPs can enhance drug-loading capacity and control the rate at which the drugs are released. AuNP hybrids with the multi-block copolymers increased the loading capacity of rifampicin and the drug’s half-life to 240 h. By sustaining the drug in the system for that long, ensured slow release of rifampicin from AuNPs at the target sites after oral administration of the AuNP conjugates to rats for 15 days. The drug on the surface was released within 24 h followed by the drug trapped in the polymer matrix after 100 h. And lastly, the drug entrapped between the AuNPs and the polymer matrix took over 240 h to be released in the interstitial space [153].
The AuNP hybrids also allow for the conjugation of multiple molecules with independent but synergistic functions. This was demonstrated by co-functionalization of the AuNPs with antimicrobial peptide (LL37) and the pcDNA that encode for pro-angiogenic factor (vascular endothelial growth factor, VEGF) and used in the treatment of MRSA-infected diabetic wounds in mice [132]. The AuNPs served dual functions, as a vehicle for the biomolecules, and also as transfection agent for the pcDNA. After topical application of the AuNP conjugates on the wound, the LL37 reduced MRSA colonies, while the pcDNA promoted wound healing by inducing angiogenesis through the expression of VEGF [132].
AuNPs have been shown to confer activity and repurpose some non-antibiotic drugs toward antimicrobial activity. The examples of repurposed drugs, which were used for the treatment of diseases other than bacterial infections, include 5FU [58], metformin [147] and 4,6-diamino-2-pyrimidinethiol (DAPT) [13, 112]. AuNPs as drug carriers are able to transport the drugs into the cells and allow direct contact with cellular organelles that resulted in their death [58, 147]. 5FU is an anti-leukemic drug, when attached to AuNPs was shown to kill some bacterial (Micrococcus luteus , S. aureus , P. aeruginosa , E. coli ) and fungal (Aspergillus fumigatus , Aspergillus niger ) strains [58]. While bacteria are resistant to DAPT, DAPT-AuNPs displayed differential antibacterial activity against the Gram-negative bacteria. Furthermore, conjugation of non-antibiotic drugs (e.g., guanidine, metformin, 1-(3-chlorophenyl)biguanide, chloroquine diphosphate, acetylcholine chloride, and melamine) as co-ligands with DAPT on AuNPs exerted non-selective antibacterial activity and a two–fourfold increased activity against Gram-negative bacteria [13]. When used in vivo, orally ingested DAPT-AuNPs showed better protection by increasing the intestinal microflora in E. coli -infected mice. After 4 weeks of treatment, the DAPT-AuNPs cleared the E. coli infection with no sign of mitochondrial damage, inflammation (increase in firmicutes ) or metabolic disorders (reduction in bacteroidetes ) in the mice [112].
The virucidal effects of the AuNP-based systems have been reported against several infectious diseases caused by influenza, measles [154], dengue [155, 156] and human immunodeficiency [115] viruses. Their anti-viral activity was attributed to the ability of AuNPs to either deliver anti-viral agents, or the ability to transform inactive molecules into virucidal agents [154, 156]. AuNPs synthesized using garlic water extracts inhibited measles viral growth in Vero cells infected with the measles virus. When the cells were exposed to both the virus and AuNPs at the same time, they blocked infection of Vero cells by the measles virus [154]. The AuNPs were nontoxic to the Vero cells up to a concentration of 100 µg/mL but inhibited viral uptake by 50% within 15–30 min at a concentration of 8.8 μg/mL [154]. Based on the Plaque Formation Unit assay, the viral load was reduced by 92% after 6 h exposure to 8.8 μg/mL of the AuNPs. The AuNPs interacted with the virus directly and blocked its transmission into the cells [154]. Modification of the AuNP surface with ligands that bind to the virus [156] or anti-viral agents [115, 155] protected them from degradation, enhanced their uptake and delivery onto the cells. The charge of the AuNPs also played a role, with cationic AuNPs being more effective in the delivery and efficacy of the AuNPs than the anionic and neutrally charged NPs. Cationic AuNPs complexed with siRNA inhibited dengue virus-2 replication in dengue virus-2-infected Vero and HepG-2 cells and also the virus infection following pre-treatment of the virus with AuNPs [155]. Inactive molecules are transformed into highly potent anti-viral agents after conjugation to AuNPs. One such example is the transformation of SDC-1721 peptide, a derivative of TAK-779, which is an antagonist of CCR5 and CXCR3 receptors for HIV-1 strain. SDC-1721 has no activity against the HIV-1, but when conjugated to the AuNPs it inhibited HIV-1 infection of the human phytohemagglutinin-stimulated peripheral blood mononuclear cells. The inhibitory effects of SDC-1721-AuNPs were comparable to the TAK-779 [115].
AuNPs as PT Agents
Diseased cells are sensitive to temperatures above 40 °C; cancer cells in particular appear to be even more sensitive to these high temperatures. Studies have shown that high fevers in cancer patients either reduced the symptoms of cancer or completely eradicated the tumors as a result of erysipelas infections [33, 157, 158]. Historically, fevers induced by bacterial infections, hot desert sand bath, or hot baths were used to increase the body temperature in order to kill the cancer cells [157]. These findings gave birth to PT therapy (PTT), which is mostly used for the treatment of cancer. PTT makes use of organic photosensitizers (indocyanine green, phthalocyanine, heptamethine cyanine) that are irradiated by the external source to generate heat energy that will increase the temperature to 40–45 °C (hyperthermia) in the target cells. Hyperthermia then triggers a chain of events (such as cell lysis, denaturation of the genetic materials and proteins), resulting in the destruction of the diseased cells [57, 158,159,160].
The organic dyes are used alone, or in combination with chemotherapy and radiotherapy for enhanced efficacy [157, 160]. Ideally, the effects of the PT agents must be confined to target cells and display minimal bystander effects. However, the organic PT dyes have several limitations such as toxic bystander effects, susceptibility to photobleaching and biodegradation [159]. In recent years, AuNPs are being explored as alternative PT agents as they exhibit strong plasmonic PT properties, and depending on their shape, they can absorb visible or NIR light. Absorption of light in the NIR spectrum is an added advantage that can allow deep tissue PTT [158, 161, 162]. Unlike organic dyes, AuNPs operate in an optical window where the absorption of light by interfering biological PT agents such as hemoglobin, melanin, cytochromes and water is very low [158, 161, 162].
The practicality of AuNP-based PTT has been demonstrated through in vitro and in vivo studies [158, 162, 163]. When the AuNPs are exposed to light, they can convert the absorbed light energy into thermal energy within picoseconds [57, 158, 159], consequently activating cell death via necrosis or apoptosis in the target cells or tissues. AuNP-based hyperthermia in diseased cells has been reported to occur at half the amount of the energy required to kill normal cells, thus perceived to be safer and better PT agents than the conventional dyes [33, 160]. AuNPs can be easily modified to have localized and enhanced PT activity by targeting and accumulating in only diseased cells through either active or passive targeting. And since the tumor environment is already hypoxic, acidic, nutrient starved and have leaky vasculature, the tumors will be most sensitive to the AuNP-based hyperthermia than the surrounding healthy cells and tissues [33, 160].
AuNP-based PTT has been extensively studied [158, 161, 162] and established that AuNPs (e.g., AuNRs, nanocages and nanoshells) that absorb light in the NIR spectrum are best for in vivo and deep tissue PTT [161]. While the ones that absorb and emit light in the visible spectrum (AuNSs and hollow AuNPs) have been demonstrated to treat diseases that affect shallow tissues (up to a depth of 1 mm), which could be of benefit to superficial tumors [158, 161, 162], ocular surgery [164, 165], focal therapy and vocal cord surgery [158, 165]. Although the PTT effects of AuNSs are limited in vivo or for use in deep tissues, combination therapy or active targeting can be incorporated to facilitate target-specific effects [158, 161, 163]. The AuNPs in the combination therapy will serve dual functions as both drug sensitizer and a PT agent, and was shown to enhance anticancer effects of chemotherapeutic drugs [158, 162, 163]. AuNS-Dox combination demonstrated enhanced cancer cell death after laser exposure when compared to the individual effects of the AuNSs and Dox with and without laser treatment [158].
Active targeting on its own can also improve AuNP uptake, localization and target-specific PT effects, which can be viewed in real time by adding fluorophores. AuNSs (25 nm) loaded with transferrin targeting molecules and FITC were shown to accumulate and destroy human breast cancer cells at a higher rate than in non-cancer cells and had better efficacy than the untargeted AuNSs [57]. An independent study also demonstrated that DNA aptamers (As42)-loaded AuNSs (As42-AuNP) induced selective necrosis in Ehrlich carcinoma cells that express HSPA8 protein, a receptor for the aptamers. None of these effects were observed in blood and liver cells mixed with target cells, or cells treated with the AuNSs without laser treatment [163]. The PT effects of the As42-AuNP were replicated in mice transplanted with Ehrlich carcinoma cells in their right leg. As shown in Fig. 9, tail-vein injections of As42-AuNPs followed by laser irradiation resulted in targeted PT destruction of the cancer cells. The As42-AuNPs reduced tumor size in a time-dependent manner; cell death was attributed to increased temperature up to 46 °C at the tumor site. The tumor in mice treated with As42-AuNPs without laser treatment and the AuNPs conjugated with nonspecific DNA oligonucleotide continued to grow but at the lower rate compared to mice injected with PBS. This suggests that the AuNPs were also localized in the tumor [163]. In cases where AuNSs are not efficient for deep tissue PTT, other shapes such as nanocages, nanoshells and AuNRs can be used [158]. Alternately, the visible light absorption of the AuNSs can be shifted to NIR by using processes such as two-photon excitation [57].
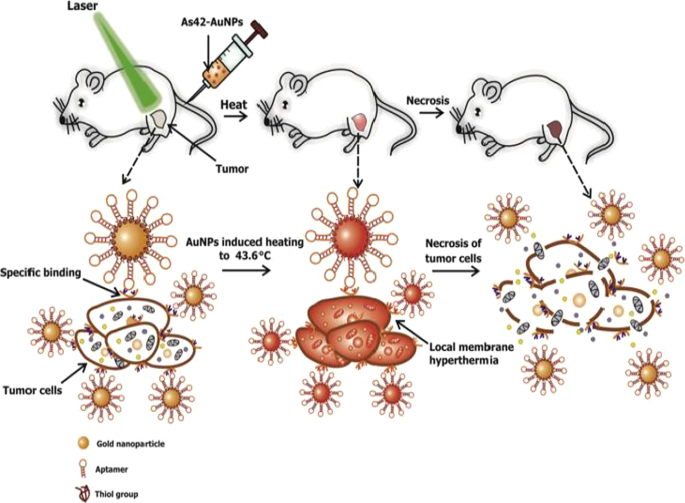
In vivo plasmonic PT therapy of cancer cells using targeted AuNSs. As42-AuNPs localized in HSPA8-expressing tumor cells after i.v injection. Exposure to laser treatment resulted in hyperthermia that caused cancer cell death. Reproduced with permission [163]. Copyright 2017, Elsevier
The PT effects of the AuNPs have also been reported for the reversal of obesity [52, 56], using hollow AuNSs (HAuNSs) [52] and AuNRs [56] for the PT lipolysis of the subcutaneous white adipose tissue (sWAT) in obese animals. The HAuNSs were modified with hyaluronate and adipocyte targeting peptide (ATP) to produce HA–HAuNS–ATP conjugate [52]. Hyaluronate was used to ensure topical entry of the HA–HAuNS–ATP through the skin [52, 166], while ATP will recognize and bind to prohibitin once the HAuNSs are internalized. Prohibitin is a receptor that is differentially expressed by the endothelial cells found in the WAT vasculature of obese subjects [5, 52, 55]. The HA–HAuNS–ATP was topically applied in the abdominal region of the obese mice, and through hyaluronate were transdermally shuttled through the epidermis into the dermis where the ATP located the sWATs (Fig. 10) . Illumination of the target site with the NIR laser selectively induced PT lipolysis of the sWAT in the obese mice and reduced their body weight [52]. The AuNRs were used in the photothermolysis-assisted liposuction of the sWATs in Yucatan mini pigs. The untargeted PEG-coated AuNRs (termed NanoLipo) were injected in the sWATs through an incision, followed by laser illumination to heat up the sWATs, which was then aspirated using liposuction. The amount of fat removed from NanoLipo-treated porcine was more than the one removed with conventional suction-assisted lipectomy (SAL). NanoLipo-assisted fat removal had several advantages over the conventional SAL; it took less time (4 min) for liposuction compared to 10 min for SAL, the swelling in the treated site healed faster, and the weight loss effects lasted over 3 months post-liposuction [56].
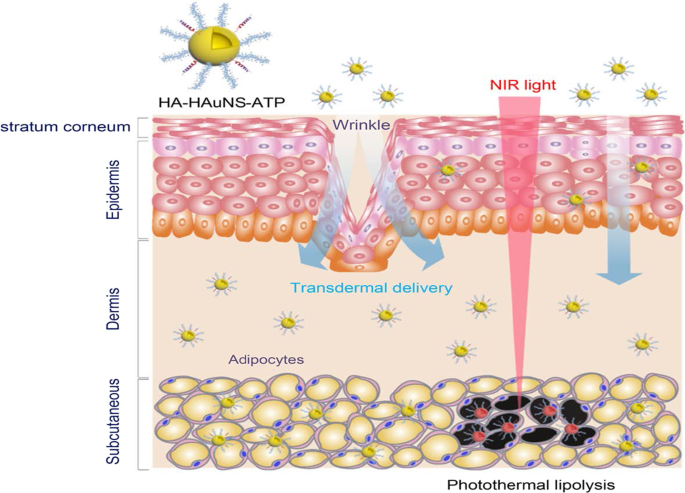
PT lipolysis of the sWATs using HA-HAuNS-ATP. The ATP was conjugated to the AuNSs for targeted delivery and destruction of the prohibitin-expressing sWATs after NIR laser exposure. Reproduced with permission [52]. Copyright 2017, American Chemical Society
AuNP-based PTT clearly offers a lot of advantages compared to the conventional agents. Their biocompatibility allows for broader applications both in vitro and in vivo. Moreover, they can be customized based on their shapes for shallow (AuNSs) [158, 161, 162] or deep tissue (AuNRs and stars) PTT [158, 161]. At 1–100 nm diameter, AuNPs and its conjugates can circulate long enough to reach and accumulate in the target tissues, with or without targeting moieties [159, 167]. Active targeting can be used to ensure localized PT effects through various routes of administration and might be effective for solid and systemic diseases. AuNP-based PTT can also be used to sensitize cancer cells when administered in combination with chemotherapy, gene therapy and immunotherapy [159]. Therefore, AuNP-based PTT has potential for treatment of chronic diseases [161].
Toxicity of AuNPs
AuNPs can play an important role in medicine, as demonstrated by the preclinical and clinical studies under review. Their full potential in clinical application as both diagnostic and therapeutic agents can only be realized if they do not pose any health and environmental hazards. While their use in vitro appears to be inconsequential, in vivo application can be hampered by their potential toxicity, which could be detrimental to human health. A major concern with their clinical use is that AuNPs are non-biodegradable and their fate in biological systems has not been fully studied [5, 30]. Although AuNPs are considered to be bio-inert and compatible, their properties (size, shape, charge and composition) raise concerns as they can alter their pharmacokinetics when used in biological environment [27, 34, 118]. The toxicity of AuNPs of varying sizes and shapes has been demonstrated in animals [27, 118]. These NPs can accumulate in the RES organs where they induce damage.
AuNPs are 1–100 nm in diameter which makes them smaller than most of the cellular components. At these sizes, AuNPs can passively transverse cellular barriers and blood vessels by taking advantage of the EPR effect in pathological cells. AuNPs with smaller diameters (1–2 nm) can easily penetrate cell membranes and biologically important cellular organelles such as mitochondria and nuclei [7, 168]. Accumulation of AuNPs in these organelles induces irreversible damage that can cause cellular demise. On the contrary, AuNPs larger than 15 nm are restricted to the cytoplasmic spaces and unable to penetrate internal organelles [168]. These features are desirable for targeting pathological cells, however, AuNPs can also be taken up by healthy cells and alter their physiology [118]. Administration of AuNP-based therapeutics can be done via different routes (i.e., intranasal, oral, transdermal, i.p or i.v) and transported through blood vessels into different tissues and organs [34, 118]. They are able to pass through the blood brain barrier and the placental barrier [34]. Toxicity is size dependent, with certain sizes of AuNPs being well tolerated, while others could be lethal to healthy tissues. Unfunctionalized AuNSs at 8, 17, 12, 37 nm caused physical changes (i.e., change the fur color, loss of bodyweight, camel-like back and crooked spine) within 14 days of treatment (2 doses of 8 mg/kg/week) in rats [118]. Most (> 50%) of the rats died within 21 days (i.e., after 3 doses), and abnormalities in the RES organs (liver, lungs and spleen) were observed. On the contrary, mice treated with 3, 5, 50 and 100 nm AuNPs were not affected by the NPs and no adverse effects or death occurred throughout the duration (50 days) of the study [118]. In diet-induced obese rats that received i.v injections of 14 nm cAuNPs, the NPs were detected in various tissues after 24 h and were mostly confined to the RES organs [55].
The shape, charge and surface chemistry of AuNPs can influence their toxicity. These factors can determine how AuNPs will interact with the biological systems, their cellular uptake and effects on the cells. AuNSs are readily taken up by cells and proven to be less toxic than other shapes such as rods and stars. AuNP surfaces are charged and will influence how they interact and behave within a biological environment [169]. Cationic AuNPs are likely to be more toxic compared to neutral and anionic AuNPs, as their charge allows these NPs to easily interact with negatively charged cell membranes and biomolecules such as DNA. Both the positively and negatively charged AuNPs have been associated with mitochondrial stress, which was not observed with the neutrally charged AuNPs [34, 35].
The shell that forms on the surface of the AuNP core can also influence the functioning of the NPs. These are usually reducing and/ or stabilizing agents such as citrate and CTAB, and once subjected to a biological environment, these molecules can cause either the desorption or absorption of biomolecules found in the biological environment. This can result in the formation of a corona or cause the NPs to become unstable. Citrate- and CTAB-capped AuNPs are highly reactive, which can facilitate the attachment of biocompatible polymers such as PEG, polyvinyl-pyrrolidone, poly (acrylic acid), poly(allylamine hydrochloride), and polyvinyl-alcohol) or biomolecules such as albumin and glutathione to prevent the formation of AuNP-corona with serum proteins. These molecules serve as a stabilizing agent and form a protective layer that can mask the AuNPs from attacks by phagocytes [7, 29, 34, 170] and prevent off-target toxicity [7]. As discussed in “AuNP-Based Therapies” section, AuNPs can be functionalized with targeting and therapeutic agents to define their targets and effects [34].
In addition to their physicochemical properties, the dosage, exposure time and environmental settings also influence the activity of AuNPs. Lower doses and short-term exposure times might render AuNP as nontoxic, while increasing these parameters will lead to cytotoxic effects [34]. Moreover, in vitro studies do not always simulate in vivo studies. At times, AuNPs that seem to be nontoxic in cell culture-based experiments end up being toxic in animal experiments. Many factors could be responsible for these discrepancies [118], and some steps have been identified that can guarantee the safety of AuNPs in biomedical applications. The biocompatibility and target specificity of AuNPs can be improved by modifying the surface of the NPs. Attaching targeting moieties on the AuNPs can channel and restrict their effects to specific targets or pathological cells [5, 55, 127]. Modification of AuNP surface with bio-active peptides provides a platform for developing multifunctional AuNPs with enhanced specificity, efficacy and potentially sustainable effects [11, 127]. All of these effects will be instrumental in the design and development of AuNP-based systems for clinical applications.
Clinical Application of AuNPs
Nanotechnology has the potential to shape the future of healthcare systems and their outcomes. Its promise of creating highly sensitive and effective nanosystems for medicine has been realized with the introduction of organic nanoformulations for cancer treatment. These systems have already paved the way for nanomaterials into clinical applications:doxil and abraxane have been in the market for over two decades and demonstrated the potential of nanotechnology in medicine [1, 2]. More recently, this technology has been used for the development of the SARS-CoV-2 lipid NP-based vaccine to fight against the COVID-19 pandemic [171]. Inorganic nanosystems such as AuNPs offer many advantages over their organic counterparts, yet few of these systems are used clinically (Table 2) [19, 32].
While several AuNP-based drugs are some of the inorganic nanomaterial-based drugs that were tested in clinical trials, they are not progressing at the same rate as organic liposome-based nanodrugs. Aurimune (CYT-6091) and aurolase were the first of AuNP-based formulations to undergo human clinical trials for the treatment of solid tumors. CYT-6091 clinical trials started in 2005 for delivery of recombinant TNF-α as an anticancer therapy in late-stage pancreatic, breast, colon, melanoma, sarcoma and lung cancer patients. CYT-6091 consists of 27-nm cAuNPs loaded with TNF-α and thiolated PEG. The CYT-6091 nanodrug has achieved safety and targeted biologic response at the tumor site at a dose lower than that required for TNF-α alone [16, 17]. CYT-6091 is approved and yet to start phase II clinical trials in combination with chemotherapy. Based on phase II clinical trial strategy, several variants of CYT-6091 have been developed and tested in preclinical studies. All the nanosystems contain TNF-α with either chemotherapy (paclitaxel, dox and gemcitabine), immunotherapy (Interferon gamma) or apoptosis inducing agents attached to the 27 nm cAuNPs [14,15,16]. The AuNP conjugates preferentially accumulated in the tumor sites after systemic administration through the EPR effect and vascular targeting effects of the TNF-α. The AuNPs were not detected in the healthy tissues, and the anti-tumor effects of TNF-α were restricted to the tumor environment [14, 16, 19].
The first clinical trial for the PT treatment with AuroLase® for refractory and/or recurrent head and neck cancers was completed. Information on the outcome of this trial is still pending. The second trial is set to evaluate the effects of AuroLase® on primary and/or metastatic lung tumors in patients where the airway is obstructed [19]. The number of human trials based on AuNP-based formulation is increasing, covering the treatment of a wide range of medical conditions including skin, oral, heart and neurological diseases. AuNP-formulation (150 nm silica-gold nanoshells coated with PEG), which is similar to AuroLase®, was approved for PT treatment of moderate-to-severe inflammatory acne vulgaris. The nanoshells were topically applied on the acne area and transdermally delivered into the follicles and sebaceous ducts through low-frequency ultrasound or massage. Nanoshells applied through massage were effective in penetrating the shallow skin infundibulum (90%) and the sebaceous gland (20%), while the low-frequency ultrasound can penetrate both shallow and deep skin tissues. NIR laser treatment resulted in focal thermolysis of the sebaceous glands in the affected area and disappearance of the acne [18, 167]. The gold–silica nanoshells were well-tolerated, showed no systemic toxic effects with minor side effects (reddiness and swelling) at the treatment site [18]. AuNPs offer many health benefits based on their unique properties but at the same time have raised a lot of political and ethical issues, and resulted in termination of some clinical studies (NCT01436123).
Conclusion and Future Perspectives
Applications of AuNPs in biomedicine are endorsed by their unique physicochemical properties and have shown great promise as theranostic agents. The increasing interest in biomedical applications of AuNPs is further encouraged by the biocompatibility and medical history of bulk gold, which suggests that the gold core in AuNPs will essentially display similar or improved properties [3]. But at the same time their small size can infer unique properties that will completely change their pharmacokinetics [144]. The diverse biomedical applications of AuNPs in diagnostics and therapeutics herein discussed demonstrate their potential to serve as adjunct theranostic agents. They can be used as drug delivery, PTT, diagnostic and molecular imaging agents [12, 33, 128]. In time, and with better knowledge of mechanisms of action, more AuNP-based systems will obtain approval for clinical use. However, the excitement of these biomedical applications of AuNPs should unequivocally be balanced with testing and validation of their safety in living systems before any clinical applications.
In conclusion, more work needs to be done to taper the toxicity of AuNPs. This can be achieved by introducing biocompatible molecules on their surface [14, 15, 58, 159], and developing new and better synthesis methods, such as the use of green chemistry to produce biogenic NPs. All these developments may further broaden the applications of AuNPs in nanomedicine. AuNPs are non-biodegradable, and off-target distribution could result in chronic and lethal effects. All these concerns must be addressed before clinical translation; the existing trials will soon provide some clarity on their impact in human health. Should their health benefits outweigh their potential risks as is the case with the existing clinical drugs, it is a matter of time before they are approved for clinical use.
Доступность данных и материалов
All the information in this paper was obtained from the studies that are already published and referenced accordingly.
Сокращения
- 5-FU:
-
5-Fluorouracil
- AD:
-
Alzheimer's disease
- ADDLs:
-
Amyloid-beta-derived diffusible ligands
- ASOs:
-
Antisense oligonucleotides
- AuNPs:
-
Gold nanoparticles
- AuNPsQ:
-
Quantum-sized AuNPs
- AuNRs:
-
Gold nanorods
- AuNSs:
-
Gold nanospheres
- BCA:
-
Bio-barcoding assay
- cAuNPs:
-
Citrate-capped AuNPs
- COVID-19:
-
Corona virus disease 2019
- CSF:
-
Cerebrospinal fluid
- CT:
-
Computed tomography
- CTAB:
-
Cetyltrimethylammonium bromide
- DABCYL:
-
4-((4′-(Dimethyl-amino)-phenyl)-azo)benzoic acid
- DAPT:
-
4,6-Diamino-2-pyrimidinethiol
- Dox:
-
Doxorubicin
- EDC:
-
1-Ethyl-3-(3-dimethylaminopropyl) carbodiimide
- EPR:
-
Enhanced permeability and retention
- FA:
-
Folate
- FDA:
-
Управление по санитарному надзору за качеством пищевых продуктов и медикаментов
- FITC:
-
Fluorescein isothiocyanate
- FRET:
-
Fluorescence resonance energy transfer
- HAuNSs:
-
Hollow AuNSs
- HF:
-
High-fat
- HU −1 :
-
Hounsfield unit
- LFAs:
-
Lateral flow assays
- LSPR:
-
Localized surface plasmon resonance
- MDR:
-
Multidrug resistant
- MGF:
-
Mangiferin
- MPA:
-
Mercaptopropionic acid
- MWPLP:
-
Microwave-induced plasma-in-liquid process
- NCL:
-
Nucleolin
- NIR:
-
Near-infrared
- NSMs:
-
Nanostructured materials
- P. jirovecii :
-
Pneumocystis jirovecii
- PEG:
-
Polyethylene glycol
- PSA:
-
Prostate-specific antigen
- PSMA:
-
Prostate-specific membrane antigen
- PT:
-
Photothermal
- PTT:
-
Photothermal therapy
- QDs:
-
Quantum dots
- RES:
-
Reticuloendothelial system
- ROS:
-
Reactive oxygen species
- SARS-CoV-2:
-
Severe acute respiratory syndrome-coronavirus-2
- SCID:
-
Severe combined immunodeficiency
- SPR:
-
Surface plasmon resonance
- sWAT:
-
Subcutaneous white adipose tissue
- TOAB:
-
Tetrabutylammonium bromide
- TRMs:
-
Tissue-resident macrophages
- VEGF:
-
Vascular endothelial growth factor
- WAT:
-
White adipose tissue
Наноматериалы
- Наночастицы золота для химиотерапевтических датчиков
- Наночастицы для терапии рака:текущий прогресс и проблемы
- Достижения и проблемы флуоресцентных наноматериалов для синтеза и биомедицинских приложений
- Графен и полимерные композиты для суперконденсаторов:обзор
- Получение наноструктуры Au @ TiO2 - оболочка и ее применение для разложения и обнаружения метиленового синего
- Модифицированный гиперразветвленный полиглицерин как диспергатор для контроля размера и стабилизации нано…
- Подготовка гибридных микроматериалов MnO2 с полипропиленовым покрытием и их улучшенные циклические характери…
- Изготовление, характеристика и цитотоксичность сферических конъюгированных наночастиц карбоната кальция, п…
- Последние достижения в синтетических методах и применении серебряных наноструктур
- Сапонины Platycodon из Platycodi Radix (Platycodon grandiflorum) для зеленого синтеза наночастиц золота и серебра