Достижения и проблемы флуоресцентных наноматериалов для синтеза и биомедицинских приложений
Аннотация
С быстрым развитием нанотехнологий в последние два десятилетия появились новые типы флуоресцентных наноматериалов (ФНМ). Нанометровый масштаб наделяет FNM уникальными оптическими свойствами, которые играют решающую роль в их приложениях в области биоимиджинга и детектирования, зависимого от флуоресценции. Однако, поскольку низкая селективность, а также низкая эффективность фотолюминесценции флуоресцентных наноматериалов в некоторой степени препятствуют их применению в визуализации и обнаружении, ученые все еще находятся в поисках синтеза новых FNM с лучшими свойствами. В этом обзоре обобщены различные флуоресцентные наночастицы, включая полупроводниковые квантовые точки, углеродные точки, углеродные наночастицы, углеродные нанотрубки, наноматериалы на основе графена, наночастицы благородных металлов, наночастицы кремнезема, люминофоры и органические каркасы. Мы подчеркиваем последние достижения последних достижений в области синтеза FNMs и их приложений в биомедицинской области за последние годы. Кроме того, были рассмотрены и обсуждены основные теории, методы и ограничения синтеза и приложений FNM. Кроме того, систематически обобщаются проблемы синтеза и биомедицинских приложений. Также представлены будущие направления и перспективы применения FNM в клинической практике.
Введение
Обычные органические красители сталкиваются с некоторыми трудностями при их применении в биомедицине из-за присущих им дефектов, таких как цитотоксичность и плохая биосовместимость [1]. Однако появление флуоресцентных наноматериалов показывает большой потенциал для замены обычных органических красителей. Ученые потратили много времени и усилий на исследования флуоресцентных наноматериалов, и соответствующие достижения в области синтеза и применения более чем вдохновляют.
Форма, размер и структура флуоресцентных наноматериалов определяют их физические и химические свойства, которые имеют огромное влияние на их характеристики. Следовательно, управляемый синтез флуоресцентных наноматериалов стал горячей темой исследований. Оптимальные экспериментальные условия синтеза способствуют наиболее подходящему размеру, морфологии и стабильности флуоресцентных наноматериалов. В последние годы были предприняты большие усилия для улучшения биосовместимости флуоресцентных наноматериалов за счет улучшения методов синтеза [2]. В прошлом ионы металлов обычно легировали углеродными точками (КТ) или квантовыми точками (КТ) для функционализации поверхности флуоресцентных наноматериалов. Однако неэффективная флуоресценция и основная токсичность представляют угрозу для их применения в биоимиджинге и биомаркировке [3]. Учитывая эти проблемы, Zuo et al. сообщили о высокоэффективной системе доставки генов CDs. CD, допированные фтором, были синтезированы с помощью сольвотермического процесса, а сайты положительного заряда для доставки генов могут быть обеспечены разветвленным полиэтиленимином (b-PEI) [4]. Можно ожидать, что новые методы модификации поверхности станут предметом исследований в будущем.
Было приложено много усилий для изучения потенциала флуоресцентных наноматериалов для биомедицинских применений, включая биоимиджинг, биодетекцию и некоторые методы терапии, как показано на рис. 1. Надежная флуоресценция для применения зависит от их физических и химических свойств [5]. Поэтому исследовательская работа по улучшению их свойств, таких как токсичность, гидрофильность и биосовместимость, была важной частью реализации широкого использования флуоресцентных наноматериалов в биомедицинских областях. С увеличением частоты некоторых заболеваний, таких как рак, возрастает потребность в новых стратегиях диагностики и терапии с более высокой точностью и соблюдением пациентом режима лечения [6]. В настоящее время легирование ионами металлов или неметаллов и модификация поверхности флуоресцентных наноматериалов по-прежнему являются доминирующими методами повышения эффективности и биосовместимости фотолюминесценции [7], и соответствующие исследования открывают новые горизонты биомедицинских применений флуоресцентных наноматериалов.
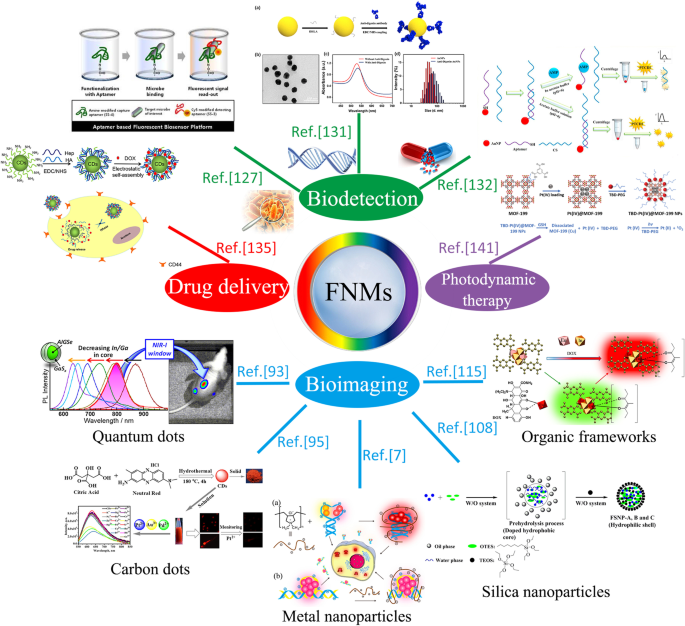
Обзорная диаграмма биомедицинского применения флуоресцентных наноматериалов
Учитывая огромный потенциал флуоресцентных наноматериалов в биомедицинской области, в этом обзоре делается акцент на последних достижениях и улучшениях. Ученые посвятили себя функционализации поверхности флуоресцентных наноматериалов и их применению в биомедицине. Только с разумно разработанными стратегиями синтеза флуоресцентные материалы могут быть наделены качеством высокой эффективности ФЛ и хорошей биосовместимостью, что имеет жизненно важное значение для их применения в биомедицинской области. Мы надеемся, что этот обзор синтеза и применения флуоресцентных материалов может помочь читателям понять общую тенденцию развития флуоресцентных наноматериалов в настоящее время.
Синтез флуоресцентных наноматериалов
Квантовые точки (полупроводниковые кристаллы)
Квантовые точки (КТ) были предметом исследований в последние десятилетия из-за их широкого спектра поглощения и симметричной фотолюминесценции, высокого квантового выхода, высокой устойчивости к фотообесцвечиванию, высоких коэффициентов молярной экстинкции и больших эффективных стоксовых сдвигов [8]. С точки зрения механизма образования квантовых точек, когда носители заряда (электроны и дырки) ограничены потенциальными барьерами в определенных областях, полупроводники демонстрируют драматические квантово-размерные эффекты, приводящие к сдвигу спектра поглощения и спектра флуоресценции. Небольшие области меньше длины волны де Бройля носителей заряда, или, что то же самое, диаметр нанокристалла менее чем в два раза больше боровского радиуса экситонов в массивном материале [9]. Когда носители заряда ограничены потенциальными барьерами в трех пространственных измерениях, образуются КТ, которые в основном состоят из атомов групп II-VI (CdSe, ZnS), III-V (GaAs, InP) или IV-VI (PbS, PbSe).
Впервые о синтезе квантовых точек сообщили в 1982 г. [10,11]. Нанокристаллы и микрокристаллы полупроводников выращивались в стеклянных матрицах. С развитием флуоресцентных материалов квантовые точки были получены различными методами, такими как метод прямой адсорбции, метод адсорбции с использованием линкера, методы на месте и комбинация предыдущих методов получения. Комбинация предыдущих методов включает комбинацию подготовленных полупроводников с предшественниками КТ и комбинацию предварительно подготовленных КТ с предшественниками полупроводников, в которой полупроводники или КТ готовятся отдельно [12].
После серии исследований по синтезу квантовых точек многие исследователи сообщили об исследовании флуоресцентных свойств квантовых точек. Bawendi et al. синтезировал КТ с узким распределением по размерам путем введения полупроводниковых прекурсоров, таких как сульфид кадмия (CdS), селенид кадмия (CdSe) или теллурид кадмия (CdTe), чтобы исследовать зависящие от размера оптические свойства КТ [13]. С тех пор CdSe стал наиболее распространенным химическим составом КТ, и для придания коллоидной стабильности использовались различные модификации поверхности [14,15,16] или защитная неорганическая оболочка [13,17].
Углеродные точки
Углеродные точки (CD) представляют собой новые наноматериалы в семействе наноуглеродов с размерами менее 10 нм, которые были впервые получены при очистке однослойных углеродных нанотрубок (ОСУНТ) с помощью электрофореза в 2004 году [18]. Примечательно, что компакт-диски постепенно замещают полупроводниковые квантовые точки на основании высокой растворимости в воде, низкой цитотоксичности, высокой фотостабильности, многоцветного излучения, зависящего от возбуждения, предпочтительной гибкости в модификации поверхности, отличной проницаемости клеток и лучшей биосовместимости [19, 20]. Как правило, компакт-диски в основном состоят из квантовых точек углерода (ККТ) и квантовых точек графена (ККТ). Множество методов синтеза компакт-дисков с регулируемым размером можно условно разделить на две основные группы:химические методы и физические методы [21].
Методы химического синтеза
Для получения углеродных точек наиболее часто используются методы химического синтеза, поскольку полученные компакт-диски обладают превосходными свойствами, такими как превосходная растворимость в воде, химическая инертность, низкая токсичность, легкость функционализации и устойчивость к фотообесцвечиванию. В общем, методы химического синтеза включают электрохимический синтез [22, 23], кислотное окисление [24, 25], гидротермальную карбонизацию [26], микроволновую / ультразвуковую обработку [27, 28, 29], методы химии растворов [30], поддерживаемый синтез [31] и др.
Среди множества синтетических методов в последние десятилетия неоднократно упоминался электрохимический синтез. Группа Чжао сообщила о новом методе получения компакт-дисков с низкой цитотоксичностью путем электроокислительного синтеза, при котором компакт-диски были получены путем окисления графитового столбчатого электрода против насыщенного каломельного электрода с помощью противоэлектрода из Pt-проволоки в NaH 2 ЗП 4 водный раствор [22]. Затем супернатант подвергали ультрафильтрации через центробежные фильтрующие устройства для получения компакт-дисков с синей и желтой флуоресценцией соответственно. Другой прямой электрохимический подход был недавно описан Qu et al. Для GQD с однородным размером 3-5 нм путем электрохимического окисления графенового электрода в фосфатном буферном растворе [23]. Фотолюминесцентный (PL) цвет этих частиц был зеленым.
Мао и др. завершил синтез компакт-дисков окислением сжиганием в 2007 году путем смешивания сажи свечи с окислителем с последующим кипячением с обратным холодильником, центрифугированием и диализом для очистки компакт-дисков. Полученные спектры фотолюминесценции компакт-дисков имеют широкий цветовой диапазон с длинами волн пиков излучения от 415 (фиолетовый) до 615 нм (оранжево-красный). Затем полученные компакт-диски дополнительно подвергали электрофорезу в полиакриламидном геле для разделения компакт-дисков с различными оптическими характеристиками. Кислотное окисление также широко используется для получения стабильных наноматериалов, таких как углеродные точки. После кислотной обработки углеродных нанотрубок / графита и кипячения с обратным холодильником полученные компакт-диски размером 3-4 нм представляли желто-коричневую прозрачную жидкость, которая испускала ярко-желтую флуоресценцию в ультрафиолетовом свете и была довольно стабильной в физиологическом растворе. Благодаря этому компакт-диски с длинноволновой (желтой / оранжевой / красной) флуоресценцией обладают лучшим проникновением. Раствор ЦД можно хранить при комнатной температуре в течение длительного времени, при этом не образуются осадки, вызывающие потерю флуоресценции [25].
Микроволновый / ультразвуковой синтез постепенно и в основном стал вспомогательной синтетической технологией в процессе синтеза [32]. Флуоресцентные компакт-диски диаметром 3-5 нм были синтезированы группой Сяо с помощью экономичного, быстрого и экологически чистого подхода с использованием микроволнового излучения [33]. Наиболее важной особенностью этого одноэтапного подхода было то, что и образование, и функционализация CD были выполнены одновременно посредством микроволнового пиролиза, полученного из ионных жидкостей, впервые [34]. Процесс реакции происходил в микроволновой печи с использованием дешевых ионных жидкостей в качестве источника углерода, и раствор менялся от бесцветного до темно-коричневого по мере прохождения реакции [35]. Tang et al. использовали ультразвуковой метод на основе глюкозы или активированного угля в качестве источника углерода для синтеза монодисперсных водорастворимых компакт-дисков. Они излучали яркую и красочную флуоресценцию [28]. Аналогичным образом Vanesa Romero et al. получили высокофлуоресцентные углеродные точки (CD), легированные азотом (N) и серой (S), после фотохимического окисления углеводов в овощах. Совместное легирование N и S увеличивает количество активных центров на поверхности CD, тем самым улучшая его люминесцентные характеристики [36]. Квантовые точки углерода, легированные азотом (NCQD), флуоресцентный зонд, были успешно применены для определения доксициклина [37]. Pathak et al. подготовили также легированные углеродные точки с азотом и серой (NSCD), которые были синтезированы из тиомочевины и трис-ацетат-этилендиаминового буфера микроволновым гидротермальным методом. NSCD были использованы для визуализации различных патогенных бактерий и клеток буккального эпителия человека благодаря многоцветной флуорометрии [38].
Учитывая, что большинство вышеупомянутых методов синтеза требовали сильной кислоты, нескольких сложных экспериментальных этапов и дальнейших модификаций с другими соединениями для улучшения водорастворимости CD и улучшения их свойств фотолюминесценции, некоторые исследовательские группы использовали гидротермальную карбонизацию фотолюминесценции углеводов, такую как хитозан, глюкоза, лимонная кислота и т. д., чтобы избежать сложных и трудоемких процессов очистки и функционализации [39]. Ян и др. описали одностадийный метод синтеза флуоресцентных компакт-дисков с высокой аминофункциональностью и квантовым выходом (QY) 7,8% путем гидротермальной карбонизации хитозана при умеренной температуре. Этот метод не требует ни сильного кислотного растворителя, ни реагента для пассивирования поверхности. Кроме того, функциональные группы на поверхности CD улучшают их растворимость в воде и снижают их потенциальную биотоксичность [26]. Многолегированные углеродные точки (MCD) с яркой и регулируемой по цвету эмиссией были синтезированы методом одного горшка без какой-либо дополнительной пассивации поверхности. Синтезированные MCD были легированы большим количеством биогенных элементов (O, N, P) и, следовательно, демонстрируют сильное флуоресцентное излучение и характеристики, зависящие от длины волны возбуждения, хорошую растворимость в воде, высокую оптическую стабильность, а также ионную стабильность. MCD могут не только избирательно и чувствительно обнаруживать Fe 3+ . в синем свете обнаружения при 15,9 нм, но также измеряют внутриклеточное Fe 3+ с помощью многоцветной флуоресцентной визуализации [40].
Для методов химии растворов окислительная конденсация арильных групп успешно применялась для получения GQD в течение последних десятилетий. Стабильные коллоидные GQD с желаемыми размерами и структурой были получены группой Ли с использованием стратегии солюбилизации. Этот метод обеспечивает возможность настройки размера и узкое распределение компакт-дисков по размерам без какого-либо непрактичного процесса разделения по размеру [30]. Что касается поддерживаемой процедуры синтеза, ряд исследовательских групп воспользовались ею для завершения синтеза монодисперсных наноматериалов, таких как наноразмерные компакт-диски. Группа Чжу использовала сферы мезопористого диоксида кремния (МС) в качестве нанореакторов, а лимонную кислоту в качестве предшественника углерода, а гидрофильные компакт-диски размером 1,5–2,5 нм были приготовлены методом пропитки. Компакт-диски с высокой эффективностью фотолюминесценции 23% были способны излучать сильную синюю люминесценцию и демонстрировать превосходные конверсионные люминесцентные свойства [31]. Ярко-желтые эмиссионные углеродные точки (Y-CD) были получены Yan et al. сольвотермическим методом с использованием безводной лимонной кислоты в качестве источника углерода и 2,3-феназиндиамина в качестве источника азота. Y-CD с большим количеством карбоксильных групп показали приличный квантовый выход флуоресценции (24%), стоксов сдвиг 188 нм, высокую чувствительность и превосходную стабильность [41]. Синтетические методы и свойства компакт-дисков представлены в таблице 1.
Физические методы синтеза
Обычно методы физического синтеза включают в себя дуговый разряд, лазерную абляцию / пассивацию и плазменную обработку. Сюй и его сотрудники окислили сажу от дугового разряда с помощью HNO 3 . и затем разделили суспензию гель-электрофорезом на ОСУНТ. Наконец, они выделили быстро движущуюся полосу наночастиц в виде углеродных точек с высокой флуоресценцией [18]. Компакт-диски с использованием наноуглеродных материалов в качестве прекурсора и экологически чистого растворителя в качестве жидкой среды были изготовлены Li et al. с помощью метода мягкой лазерной абляции [44]. Кроме того, Гокус и его сотрудники продемонстрировали, что использование кислородной плазмы может вызвать сильную флуоресценцию однослойного графена [45].
Углеродные наночастицы
Флуоресцентные углеродные наночастицы с их пониженной цитотоксичностью, устойчивостью к фотообесцвечиванию и повышенной биосовместимостью привлекают все большее внимание для биоимиджинга и других биомедицинских приложений. По сравнению с типичными размерами углеродных точек в пределах 1–6 нм размеры углеродных наночастиц превышают 20 нм, что избавляет от необходимости разделять, очищать и собирать [46]. Методы синтеза углеродных наночастиц аналогичны углеродным точкам, включая гидротермальную карбонизацию, микроволновую обработку, метод химической абляции и лазерную абляцию. Эти методы имеют свои преимущества, но не могут эффективно контролировать размер наночастиц. Электрохимическая карбонизация - это одностадийный метод, позволяющий контролировать размер и люминесцентные свойства углеродных наночастиц. К сожалению, для этого метода доступно очень мало субстратов. В настоящее время сообщается о некоторых новых интересных методах, таких как метод сжигания пятиокиси фосфора [47].
В последние годы углеродные наночастицы, подходящие для биомедицинских приложений, синтезируются модифицированными методами. Santu et al. разрешили синтез высококачественных красных флуоресцентных углеродных наночастиц путем контролируемой карбонизации резорцина [48]. Этот подход включает окислительное сочетание фенола, связанное с дегидратацией, с образованием красных флуоресцентных углеродных наночастиц. Анара и др. синтезировали флуоресцентные наночастицы углерода с квантовым выходом 6,08% модифицированным гидротермальным методом. По сравнению с традиционными методами, требующими длительной термической обработки до нескольких часов, этот метод сократил время реакции до менее 30 минут, реализовав быстрый синтез флуоресцентных углеродных наночастиц [46].
Углеродные нанотрубки
Одномерные (1D) углеродные нанотрубки привлекли огромное внимание в области биомедицины благодаря своим превосходным электронным и оптическим свойствам. Углеродные нанотрубки можно разделить на одностенные углеродные нанотрубки (ОСУНТ) и многостенные углеродные нанотрубки (МУНТ) в зависимости от количества цилиндрических слоев графена. В то время как ОУНТ состоят из одного слоя листа графена, свернутого в цилиндр, МУНТ состоят из нескольких концентрических слоев листа графена. Внешний диаметр углеродных нанотрубок составляет менее 100 нм, но их длина может достигать нескольких миллиметров, что приводит к очень высокому аспектному соотношению и большой площади поверхности [49]. Более того, уникальное расположение атомов углерода в углеродных нанотрубках формирует богатое π-электронное сопряжение вне нанотрубки [50]. Кроме того, углеродные нанотрубки обладают сильным поглощением и флуоресценцией в ближней инфракрасной области [51]. Все эти характеристики способствуют эффективному взаимодействию с биомолекулами, что делает углеродные нанотрубки идеальным кандидатом для биомедицинских приложений.
Синтетические методы имеют большое влияние на диаметр, длину, структуру, хиральность и качество углеродных нанотрубок, и в то же время следует учитывать, подходит ли этот метод для крупномасштабного производства. Обычно используемые методы включают дуговой разряд [52], лазерную абляцию [53] и химическое осаждение из газовой фазы [54]. Вдобавок к этому углеродные нанотрубки необходимо функционализировать, чтобы улучшить их растворимость и предотвратить их агрегацию в растворителях и биологических средах. Ковалентная функционализация привела бы к дефектам в структуре углеродных нанотрубок, что привело бы к резкому снижению или даже полной потере их NIR-флуоресценции. Нековалентная функционализация амфифильными молекулами, такими как полимеры, сохранит структуру и флуоресцентные свойства углеродных нанотрубок, но снизит QY углеродных нанотрубок. Чтобы преодолеть эти препятствия, недавно появились сообщения о новых методах синтеза и функционализации углеродных нанотрубок. Ли и др. сообщили, что добавление дитиотреитола, который является восстановителем, может впервые усилить флуоресцентный QY ОСУНТ, в результате чего флуорофоры имеют яркость, эквивалентную яркости КТ [55]. Hou et al. исследовали добавление дитиотреитола к ОУНТ, функционализированным различными поверхностно-активными веществами. Для ОУНТ, обернутых ДНК и SDS, флуоресцентный QY из них значительно увеличился, в то время как тушение флуоресценции в различной степени наблюдалось для других поверхностно-активных веществ [56]. В результате добавление дитиотреитола к ДНК или SWCNT, завернутым в SDS, является возможным решением для достижения применения углеродных нанотрубок в биомедицине.
Наноматериалы на основе графена
Как двумерные углеродные наноматериалы, графен и его производные широко использовались в различных биомедицинских приложениях, таких как биоимганизация и доставка лекарств. Наноматериалы графена включают нанолист графена, нанолист оксида графена (GO) и восстановленного оксида графена (rGO). У них большая площадь поверхности и уникальные свойства поверхности, которые позволяют нековалентно взаимодействовать с молекулами красителей, биомолекулами и нерастворимыми в воде лекарствами. Многие исследователи сообщали о различных методах получения графена с тех пор, как он был успешно получен впервые в 2004 году. Синтетические методы графеновых наноматериалов можно разделить на две категории:нисходящие и восходящие.
Нисходящие методы включают изоляцию от уложенных друг на друга слоев графита для формирования листов графена, включая механическое расслоение [57], расслоение на основе растворителя [58] и электрохимическое расслоение [59]. Gu et al. систематически изучали отшелушивание на основе растворителей с помощью ультразвука и обнаружили, что ультразвуковые волны обладают хорошим отшелушивающим эффектом. Они также могут влиять на распределение листов графена по размеру и толщине, что делает возможным управляемый синтез. Подходы «снизу вверх» предполагают реорганизацию атомов углерода с использованием альтернативных источников углерода. Эпитаксиальный рост [60] и химическое осаждение из паровой фазы (CVD) [61] являются наиболее распространенными методами восходящего синтеза. Листы GO, состоящие из множества sp 2 домены, изолированные кислородсодержащими группами, могут быть синтезированы с использованием метода Хаммера. Варианты размеров этих sp 2 домены делают ФЛ листов GO в широком диапазоне от 500 до 800 нм [62]. rGO получают из GO путем химического восстановления с использованием восстановителей, таких как гидрохинон и гидразин. По сравнению с GO, флуоресценция rGO показывала смещенное в синий цвет излучение в УФ-области наряду с гашением флуоресценции, которое приписывается путям перколяции между вновь образованными кристаллическими sp 2 кластеры [63]. Акбари и др. выяснил, что соотношение sp 3 / sp 2 доменов в листах GO определяет их спектры флуоресценции. Таким образом, GO представляет собой многообещающий флуоресцентный наноматериал с широким диапазоном длин волн при разной степени восстановления, который может быть использован в биомедицинских приложениях.
Металлические наноматериалы
В то же время атомы благородных металлов обладают меньшей цитотоксичностью по сравнению с квантовыми точками. Наночастицы золота, серебра и меди привлекают все большее внимание и применяются во многих областях. В биомедицине квантово-механические эффекты наночастиц золота, такие как излучение фотолюминесценции или плазмонный резонанс, делают наночастицы золота (AuNP) идеальным кандидатом для другого наносенсора in vivo с низкой цитотоксичностью [64, 65].
AuNP привлекли широкий научный интерес благодаря простоте их синтеза и уникальным свойствам, и сообщалось о различных синтетических методах. В качестве одного из наиболее важных методов химические методы обычно выполняются путем обработки водного раствора хлораурата восстанавливающими агентами в присутствии стабилизирующего агента. Чаще всего используется лимонная кислота, которая может действовать как стабилизатор, так и восстановитель [66]. Однако AuNP, стабилизированные лимонной кислотой, могут необратимо накапливаться во время развития функционализации тиолатными лигандами. Эту проблему можно решить, заставив реакцию протекать в присутствии водорастворимых полимеров, поверхностно-активных веществ или покрывающих агентов, которые помогают обеспечить более высокую стабильность и предотвратить агрегацию наночастиц. Размер и форму AuNP можно контролировать, изменяя соотношение золота и цитрата, агентов, модифицирующих поверхность, или условий реакции. С помощью метода ультразвукового эмульгирования в одном сосуде Чжан и его коллеги совместно загрузили бис (4- (N- (2-нафтил) фениламино) фенил) -фумаронитрил и AuNP в мицеллы для получения нанозонда [67]. Прежде всего, полученный нанозонд с большим потенциалом для применения в визуализации опухолей и диагностике in vivo, несмотря на наличие наночастиц золота, обладал превосходными характеристиками флуоресцентной визуализации. Хотя AuNP нетоксичны в определенных экспериментальных условиях, их токсичность и побочные эффекты необходимо тщательно изучить [68].
Флуоресцентным нанокластерам Ag уделяется большое внимание из-за их уникальных физических и химических свойств. Процесс синтеза таких нанокластеров классифицируется по стабилизирующему каркасу на олигонуклеотиды, пептиды, белки, дендримеры и полимеры ДНК. Кроме того, обширная литература продемонстрировала некоторый зеленый синтез, такой как применение водных экстрактов стеблей D. trifoliata и S. alba для оптимизации условий приготовления [69].
Нанокластеры Cu (НК Cu) относительно широко используются в качестве материалов из благородных металлов, но их синтез все еще недостаточен из-за их уязвимости к окислению. Недавно Кавасаки и др. успешно получены стабильные НК Cu с помощью полиольного метода с помощью микроволнового излучения [70]. ДНК может быть использована в качестве матрицы для синтеза флуоресцентных НК Cu. Mohir et al. предложил метод, основанный на использовании двухцепочечной ДНК в растворе, для получения НК Cu с высокой селективностью [71]. Используя флуоресцентные свойства НК Cu, он был успешно использован в качестве эффективного флуоресцентного индикатора сигнала включения для селективного определения гексогена [72].
Наночастицы кремнезема
Принимая во внимание свойства прозрачности, механической стабильности, прочности и стабилизации встроенных флуорофоров, наночастицы кремнезема широко применяются в биологических областях. Например, наночастицы из диоксида кремния с ядром / оболочкой, применяемые для внутриклеточного обнаружения Zn 2+ и H 2 ЗП 4 - в живых клетках были синтезированы как «вкл / выкл» флуоресцентные наносенсоры. В последние годы наночастицы кремнезема, легированные органическими красителями, были синтезированы и широко используются во многих приложениях, таких как биодетекция [73]. Наиболее широко применяемыми методами синтеза наночастиц кремнезема являются метод Штёбера и метод обратной микроэмульсии. Метод Штёбера, впервые описанный в 1960-х годах [74], включает гидролиз алкилсиликатов и последующую конденсацию кремниевой кислоты в спиртовых растворах, катализируемую добавлением аммиака. Второй метод образования наночастиц кремнезема, метод обратной микроэмульсии, включает реакцию алкилсиликатов, обычно TEOS, внутри капель воды микроэмульсии вода-в-масле [75]. He et al. подготовил три вида красителя, легируя наночастицы диоксида кремния с помощью метода Штёбера и метода обратной микроэмульсии, встраивая краситель в ядро частицы. Некоторые молекулы функционально реагируют на Zn 2+ осаждаются на поверхности частиц [76]. Флуоресцентные наночастицы кремнезема были использованы для флуоресцентных изображений внутриклеточного Zn 2+ (H 2 ЗП 4 - ) в клетках HeLa. Когда Zn 2+ добавлен пропорциональный Zn 2+ наносенсор, наночастицы показали способность ратиометрически определять концентрацию H 2 ЗП 4 - .
В общем, нанокомпозиты на основе диоксида кремния с хорошей монодисперсностью и биосовместимостью могут быть легко модифицированы функциональными группами [77,78,79]. Ли и др. легированные магнитные наночастицы и флуоресцентные красители в наночастицы кремнезема. Эти нанокомпозиты на основе диоксида кремния могут использоваться не только в качестве зондов для мультимодальной визуализации для магнитно-резонансной (МР) и флуоресцентной визуализации, но и в качестве носителей для доставки противоопухолевых лекарств [80]. Короче говоря, частицы кремнезема могут стать предметом исследования в таких случаях с очень широким использованием.
Люминофоры
Люминофоры широко используются в биомедицине благодаря их уникальным преимуществам в снижении автофлуоресценции и светорассеивающих помех тканями. Обычно люминофоры состоят из материалов-хозяев и легированных ионов [81]. Среди основных материалов люминофоров оксид иттрия (Y 2 О 3 ) более чем многообещающе из-за его низкой фотостойкости и фононной энергии. Лантаноиды широко используются в люминофорах, учитывая их обильные уровни электронов и каналы передачи энергии. Сообщалось о многочисленных методах получения люминофоров, включая гидротермальный [82], пиролиз с распылением пламенем [83], золь-гель [84] и процессы соосаждения [85].
Гидротермальный синтез становится идеальным процессом, который доказал свою эффективность и экономичность для синтеза люминофоров. Yu et al. синтезированный Y 2 О 3 :Eu 3+ люминофоры гидротермальным методом в присутствии цитрата натрия [82]. Концентрат цитрата натрия, добавленное количество NaOH и Eu в гидротермальном процессе определили свойства полученных люминофоров. Пиролиз распылением пламени - перспективный метод быстрого и последовательного синтеза люминофоров на основе оксидов. По сравнению с обычными методами этот метод обеспечивает люминофоры с высокой степенью кристалличности и однородным распределением примесей. Хан и др. успешно произведено Tb 3+ –Допированный Y 2 О 3 люминофоры диаметром около 100 нм с узким распределением по размерам с использованием пламенного пиролиза [83]. В их методе щелочная соль смешивалась с предшественниками нитратов других металлов, что эффективно контролировало распределение по размерам в узком диапазоне. Путь золь-гель синтеза предлагает несколько преимуществ, таких как высокая гомогенность и чистота, сокращение времени синтеза, однородная морфология частиц и узкое распределение частиц по размерам [86]. Леонардо и др. получил Sm 3+ легированный SiO 2 -Gd 2 О 3 люминофоры золь-гель методом [84]. Co-precipitation is a common and simple method for synthesizing crystalline phosphors, which ensures high homogeneity and controlled morphology characteristics. Perhaita et al. reported that the phase composition of the phosphors strongly depends on the pH during the precipitation [85].
Organic Frameworks
Covalent-organic frameworks (COFs) are new porous crystalline materials possessing outstanding stability, adsorption and low toxicity. The design of fluorescent small organic molecules with a combination of fluorescence determination methods can be used to construct more efficient nanoprobes [87]. For selective 2,4,6-trinitrophenol (TNP) determination, a novel Naphthalimide-Benzothiazole conjugate was prepared as colorimetric and fluorescent nanoprobe. The fluorescence emission peaks of receptor were selectively quenched by TNP with a limit of detection as low as 1.613 × 10 –10 M.
Metal organic frameworks (MOFs) are a kind of new generation multifunctional inorganic–organic materials with various holes and functionalized 3D crystalline structures formed by metal ions and linkers. MOFs show potential applications in separation, catalysis and other aspects due to unique attributes such as excellent chemical tenability, specific surface area and confinement of the pores. Some of the MOFs are luminescent and the quantum yield as well as light intensity will be influenced by temperature and excitation wavelength [88]. With the addition of doxycycline, Yu et al. synthesized a new functional metal–organic framework of pyromellitic acid and europium, which exhibited remarkable fluorescence enhancement at 526 nm and 617 nm. Results showed that both fluorescence intensities were positively correlated with the doxycycline concentration. The unique fluorescence response of the system could discriminate doxycycline from other tetracycline antibiotics with high selectivity.
Biomedical Applications of Fluorescent Nanomaterials
Bioimaging
Quantum Dots for Bioimaging
Fluorescent nanomaterials have been widely used in bioimaging. Compared with conventional organic fluorescent molecules, fluorescent nanomaterials are equipped with many superior properties such as high photostability, tunable emission spectra and high quantum yields [89].
As early as 1998, QDs were first successfully applied in biological imaging [90]. Since then, applications of QDs in this field have been springing up gradually. Chen’s group applied it in bioimaging and nuclear targeting with great stability and biocompatibility in living cells [91]. In spite of the extremely high sensitivity and spatial resolution of QDs, poor performances on hydrophilicity and biocompatibility hindered their applications in bioimaging in vivo. To tackle this problem, it has been found that the water-solubility of QDs can be greatly improved by attaching thiol or other hydrophilic groups to the surface of quantum dots [92]. In the same way, with the intention of improving the effectiveness and specificity of in vivo targeted imaging, targeting molecules are attached to the surface of QDs. Furthermore, the wavelength region of the emission light can be controlled by altering the size of QDs.
The combination of QDs and inorganic metal ions can optimize the application of QDs in bioimaging because the QDs’ defect-site PL peaks will be utterly removed by controlling the proportion of doped inorganic metal ions. Kuwabata, S et al. modulated the degree of Ga 3+ doping in Ag–In–Se QDs. Thus, the QDs’ defect-site PL peaks were completely removed and a sharp band-edge emission peak come into appearance [93]. They found a blue shift of the band-edge PL peak ranging from 890 to 630 nm which could be credited to the fact that the energy gap of QDs was enlarged by Ga 3+ doping. After injecting a mouse with QDs, the potential of AIGSe@GaSx core–shell QDs for bioimaging turned out satisfying. The imaging effect of this kind of QDs in mice is demonstrated in Fig. 2. However, sensing of mid-IR wavelengths is challenging due to increased dark currents and noise. HgTe QDs synthesized by colloidal method is a promising candidate for IR bioimaging by virtue of lower dark currents, higher-temperature operation, and higher detectivity [94].
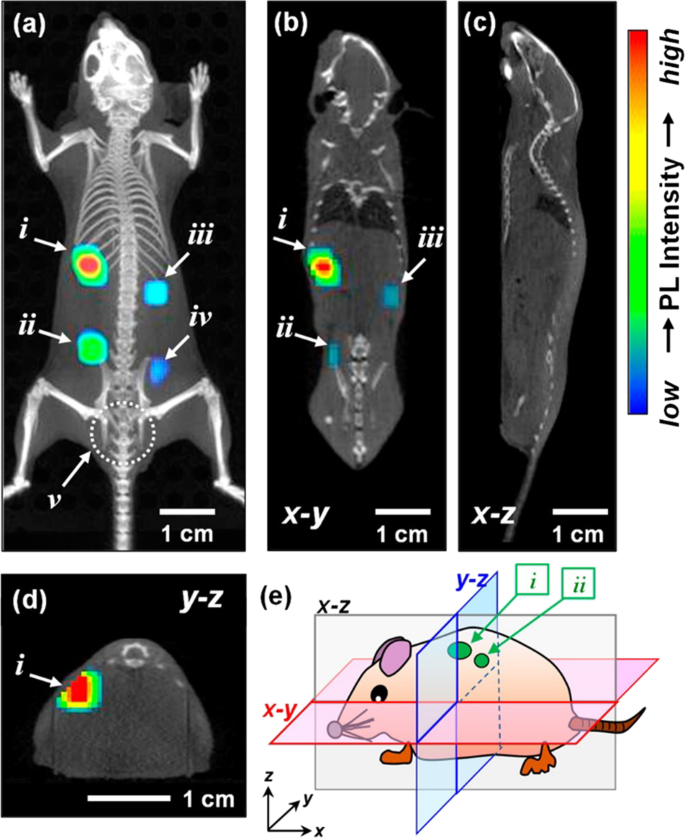
Three-dimensional PL image superimposed on an X-ray CT image of the mouse subcutaneously injected with DSPC-AIGSe@GaSx liposome dispersions (each 50 mm 3 ) in the back [78]
Carbon Dots for Bioimaging
The poor photostability of current fluorescent nanomaterials hinders their long-term bioimaging to a large extent. To overcome this limitation, CDs have been studied for bioimaging and some positive results have been obtained due to the great performance on PL efficiency. Enormous efforts have been put to improve their water solubility and lower their toxicity in organisms. At present, most CDs are facing a barrier in bioimaging, that is, their short-wavelength excitation disables deep penetration in tissue. Aside from this, being exposed under the short-wavelength for a long time could do irreversible damages to living cells and tissues. As shown in Fig. 3, with the purpose of overcoming this deficiency, Gao et al. designed fluorescent CDs with red emission which were successfully used for bioimaging of noble metal ions (Pt 2+ , Au 3+ , Pd 2+ ) in cells and zebrafish [95]. Sun and co-workers first studied the near infrared (NIR) imaging of CDs in vivo using mice as a model. Recently, it was reported that molecules or polymers containing plentiful sulfoxide or carbonyl groups can enhance NIR fluorescence through the surface modification. As shown in Fig. 4, under NIR excitation, sulfoxide or carbonyl groups are bound to the outer layers and the edges of the CDs. Thus, electron transitions are promoted, influencing the optical bandgap [96].
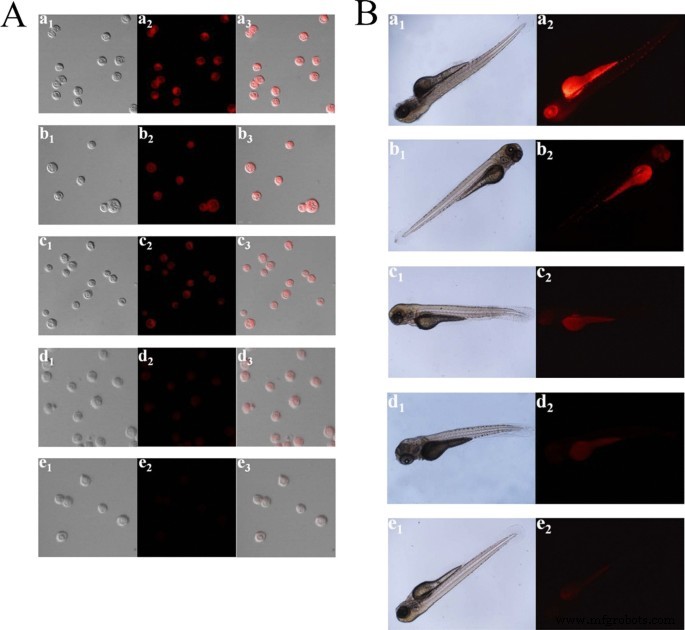
A Confocal imaging of Pt 2+ in PC12 cells. (a1–e1) Bright field images. (a2–e2) Black field images of the CDs in PC12 cells with the different concentrations of Pt 2+ (0, 25, 50, 150, and 300 μM). (a3–e3) Overlay images. B Fluorescence imaging of Pt 2+ in ZF. (a1–e1) Bright field images. (a2–e2) Fluorescence images of the CDs in ZF with the various concentrations of Pt 2+ (0, 30, 60, 100, and 150 μM) [80]
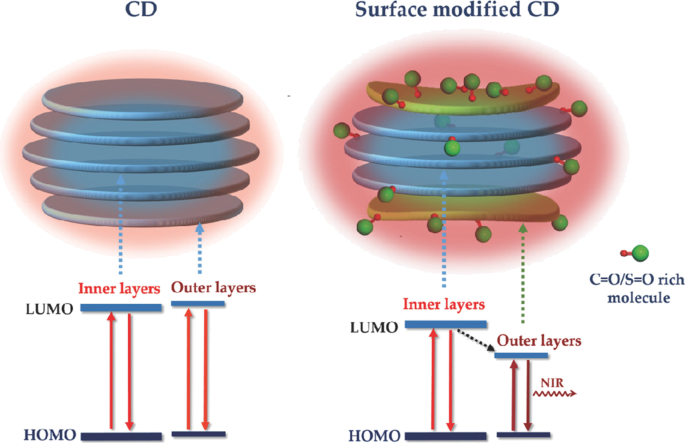
Schematic of structure and energy level alignments of nontreated CDs (left column) and CDs modified with S = O/C = O‐rich molecules (right column). The red (oxygen atom) and green double‐bonded balls represent the C = O/S = O‐rich molecule [81]
Carbon Nanoparticles for Bioimaging
In the field of bioimaging, fluorescent carbon nanoparticles show unique chemical and optical properties over traditional fluorescence probes. Different size, shape and elemental composition make carbon nanoparticles with different features. The biomedical fields are always seeking the most promising fluorescent carbon nanoparticles. Gaurav et al. obtained both larger and smaller size carbon nanoparticles with laser ablation method [97]. Both green and blue fluorescence were observed in the cells incubated with the carbon nanoparticles, suggesting their different sizes. Cell viability results indicated that the prepared carbon nanoparticles were nontoxic and safe for bioimaging applications. Shazid et al. employed carbonization method to obtain fluorescenct carbon nanoparticles derived from biocompatible hyaluronic acid. Both the in vitro and in vivo bioimaging studies showed that the prepared carbon nanoparticles would be reliable and stable for opticle imaging. Moreover, based on the experimenal data, their cytotoxicity was proved to be tolerable for biomedical applications.
Carbon Nanotubes for Bioimaging
Fluorescence of carbon nanotubes in the NIR is attracting high attention for their good light penetration depth in biological tissues. However, their low quantum yield requires for considerable excitation doses, leading to a fair degree of blue-shift and failure of penetrating live tissue. Mandal et al. reported that bright and biocompatible p-nitroaryl functionalized SWCNTs, encapsulated in phospholipid-polyethylene glycol, are suitable for bioimaging applications. The prepared SWCNTs enabled high signal-to-noise ratio imaging in live brain tissues using ultra-low excitation intensities. Their 1160 nm emissions in the NIR guarantee that they will provide optimal fluorescence imaging results [98]. Ceppi et al. applied SWCNT-based fluorescence imaging to debulking surgery in an ovarian cancer mouse model. SWCNTs are coupled to an M13 bacteriophage carrying modified peptide binding to the SPARC protein, which is overexpressed in ovarian cancer, leading to real-time imaging to guide intraoperative tumor debulking. This imaging system enables detection in the NIR window with a pixel-limited resolution of 200 μm, demonstrating real potential in fluorescence imaging guided surguries for patients [99].
Furthermore, fluorescent moieties can be conjugated by a carbon nanotube backbone, which integrate strong fluorescent ability with robust mechanism strength, exhibiting ideal bioimgaing results. Katharina et al. functionalized SWCNTs with an amphiphilic C18 -alkylated polymer conjugated with bright perylene bisimide fluorophores. The polymers wrapping around the SWCNT backbones not only increase their water dispersibility but also promote their biocompatibility by providing a shield. In vitro studies on HeLa cells demonstrated that the biocompatibility of SWCNTs is dramatically improved. In microscopy studies, direct imaging of the SWCNTs' cellular uptake via perylene bisimide and SWCNT emission proved their potential for bioimging [100]. Park et al. combined carbon nanotubes with mussel adhesive proteins which can be specifically targeted at tumors in tissue. They then made carbon nanotubes conjugated with a ZW800 NIR fluorophore to obtain NIR fluorescence imaging [101]. The prepared carbon nanotube probes react with a specific tumor in one hour and can be easily eliminated via urine, demonstrating great value as tumor imaging and detecting agent.
Graphene-Based Nanomaterials for Bioimaging
Large surface area and feasible further functionalization make graphene-based nanomaterials a promising candidate for biomedical applications. However, as a result of their chemical sturctures, graphene nanosheets lack photoluminescence and rGO only display weak fluorescence, which makes it difficult to be utilized in bioimgaing applications. Many researchers attempted to resolve this problem by conjugation of fluorescent dyes and probes onto the large surface of graphene and its derivatives. Sun et al. reported an assembly strategy to prepare fluorescence probe RACD functionalized a single layer GO via π-π interaction and hydrogen bonding. The resluting nanomaterials exhibited that the fluorescent probes reduce the aggregation degree and acquire very well monodispersion, hydrophilicity and photostability, which is attributed to the strong synergy between RACD and GO [102]. Even so, fluorescence quenching remains a critical issue for these materials. In addition, the biocompatibility and toxicity of polymers applied to connect graphene-based materials and fluorescent moieties have not been adequately investigated. These facts appeal for alternative solutions to utilize graphene and its derivatives in bioimaging applications. Georgia et al. developed intrinsically photoluminescent graphene derivatives that show desirable biocompatibility and tunable fluorescence properties [103]. They can be organophilic or hydrophilic with different amine functionalization dodecylamine and hexamethylenediamine, respectively. The intrinsic fluorescent graphene-based nanomaterials possess great potential in a variety fileds of bioimgaing.
Metal Nanomaterials for Bioimaging
In recent years, fluorescent metal nanoparticles have shown great potential in bioimaging for improved disease diagnosis and treatment [104]. Gold is the most commonly used metal for bioimaging. The surface of AuNPs can be easily modified with various biomolecules such as peptides, proteins, antibodies, enzymes, and nucleic acids. These biomolecules can interact with specific cells or organelles in vivo, which makes it possible for AuNPs to be used for targeted optical imaging. Gao et al. reported a real-time in situ imaging of nucleus by AuNPs fabricated with bifunctional peptides constructed with both Au-binding affinity and nucleus-targeting ability. The bifunctional peptides showed strong binding affinity toward AuNPs and ensured good surface coverage of the nanoparticles, which made it stable and efficient for precise bioimaging of the nucleus in cells [105]. The Au-Se bond is considered as a better candidate than the Au–S bond to link the peptides and AuNPs due to the stronger ability against interference of intracellular thiol. Pan et al. prepared the Au-Se-peptide nanoprobes through a direct freezing process. The obtained nanoprobe was successfully applied to identify autophagy and apoptosis in chemotherapeutic drug treated cancer cells [106].
As a novel fluorescent imaging technology, DNA-templated silver nanoclusters (DNA-Ag NCs) have aroused the attention of many scientists due to their unique properties, especially the tunable fluorescence emission range relying on DNA sequences. However, the highly negatively charged DNA backbones have always been a great obstacle for the expansive applications in bioimaging because of poor stability as well as poor cell permeability in physiological environment. It is also noteworthy that the PL property and fluorescent efficiency of DNA-Ag NCs are far from satisfying. As a result, figuring out how to neutralize the negative charge on the surface of DNA strands is of great urgency for researchers. Recently, Lyu and co-workers successfully modified fluorescent DNA-Ag NCs with cationic polyelectrolytes via electrostatic force between the positively charged polyelectrolytes and the negatively charged phosphate groups of the DNA strands, leading to a threefold fluorescence intensity enhancement [7] (Fig. 5). Li et al. reported a facile strategy to make gold nanoclusters with positive charge and silver nanoclusters with negative charge form aggregates by electrostatic interactions. An incredible 40-fold fluorescence intensity enhancement was obtained. Results demonstrated that the physiological stability improved a lot and the cell permeability was also enhanced, which promises its practical applications in the future.
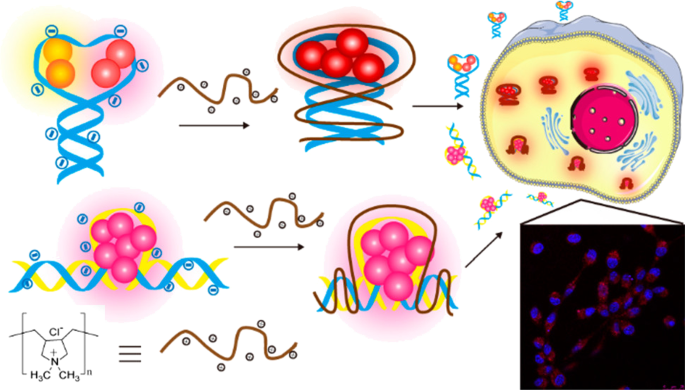
Formation of FL DNA–Ag NC–Cationic Polyelectrolyte Complexes for Cell Imaging [7]
Silica Nanoparticles for Bioimaging
Dye-doped fluorescent silica nanoparticles emerge with great potential for bioimaging as a novel and ideal platform for the monitoring of living cells and the whole body. The outer silica shell matrix protects fluorophores from outside chemical reaction factors as well as provides a hydrophilic shell for the inside insoluble nanoparticles, which renders the enhanced photo-stability and biocompatibility to the organic fluorescent dyes. Benefiting from the robust structure of silica matrices, dye-doped fluorescent silica nanoparticles have been presented with several superior properties including good biocompatibility, hydrophilic features, and high fluorescence intensity [107].
Jiao et al. also constructed a local hydrophobic cage in dye-doped fluorescent silica nanoparticles to improve their optical properties, which solves the problems of aggregation-caused quenching (ACQ) and poor photostability in aqueous media by organic fluorescence dyes benefiting from the robust structure of silica nanoparticles [108]. In addition, compared with free dyes, the fluorescent intensity both in water solution and living cells demonstrated a 12.3-fold enhancement due to the limitation of molecular motion, indicating a significant development for silica nanoparticles in biomedical applications. QDs have been developed for bioimaging both in vivo and vitro owing to their excellent optical qualities. However, a critical obstacle faced in QDs’ application in vivo is their poor biocompatibility. Inspired by the organic dye-conjugated silica-NPs, QDs-embedded silica-NPs have also been invented with the advantage that the excellent optical qualities of QDs can be retained, while the silica-NPs coat improves their biocompatibility to a large extent simultaneously. Darwish et al. reported that many QDs could be assembled around a central silica nanoparticle to form supra-NP assemblies. It was expected to be used for enhanced bioimaging because of their higher sensitivity and superior signal-to-background ratios [109]. There is reason to believe that silica-NPs conjugated with fluorescent nanomaterials with ideal optical properties will still be the dominant research interest in the future.
Phosphors for Bioimaging
For bioimaging, the sizes of phosphors need to be controlled so that they are small enough to be integrated with living cells. Furthermore, the aggregation of particles should be avoided for biocompatibility. Hence, the control of both particle sizes and dispersity in an aqueous solution is essential for the bioimaging application of the phosphors. Atabaev et al. prepared Eu, Gd-codoped Y2 O3 phosphors which had a spherical morphology within the range 61–69 nm. Enhanced PL emission and low toxicity made these phosphors suitable for bioimgaing applications [110].
Upconversion nanomaterials are able to convert lower-energy near-infrared photons to higher-energy ones as emission. This anti-Stokes photoluminescence process will lead to low background noise, large tissue penetration depth, and low photo-damage in bioimaging applications [111]. Lanthanide-based phosphors are able to show upconversion emission owing to their photodurability and low phonon energy. Nallusamy et al. reported a NIR–NIR bioimaging system based on Er 3+ :Y2 O3 phosphors by using NIR emission at 1550 nm under 980 nm excitation, which can allow a deeper penetration depth into biological tissues than ultraviolet or visible light excitation [112]. In addition, the surface of Er 3+ :Y2 O3 was electrostatically PEGylated to improve the chemical durability and dispersion stability under physiological conditions. Thakur et al. synthesized Ho 3+ /Yb 3+ co-doped GdVO4 phosphors via a modified sol–gel method. The prepared phosphors showed brilliant red upconversion emission under NIR excitation, which may be useful in bioimaging of the biomolecules [113].
Organic Frameworks for Bioimaging
Careful selection of MOF constituents can yield crystals of ultrahigh porosity and high thermal and chemical stability, with some of them being luminescent [114]. Recently, Sava Gallis’s group described a novel multifunctional MOF material platform which showed a wide spectral region from 614 to 1350 nm covering the deep red to NIR region. Both porosity and tunable emission properties made them highly suitable for in vivo bioimaging [115]. What’s more, to overcome the obstacle of MOF’s low selectivity towards malignant tissues, Liu et al. developed a target-induced bioimaging by conjugating DNA aptamers using ZrMOF nanoparticles as quenchers [116]. Based on the quenching of ZrMOF nanoparticles, target-induced bioimaging is achieved upon binding with the target.
Biodetection
Since fluorescent nanomaterials can amplify the fluorescent signals significantly and be compatible with organisms, there is increasingly more research on their application in the rapid detection of biomolecules [117]. It will shorten the analysis time to a large extent if we are able to establish a real-time detection system by fluorescent nanomaterials. It has been discovered that multiple detection can be achieved by using QDs probes simultaneously [118, 119].
Pathogen Detection
Pathogens have been an unignorable threat to human health for centuries and these include many types of microorganisms ranging from bacteria (pathogenic Escherichia coli, Salmonella, and Streptococcus pneumoniae ) and viruses (Coronavirus, Influenza virus and hepatitis virus ). However, conventional methods for pathogen detection still need improvement of detection limits and detection speeds. For their applications in detecting pathogens, Tan and co-workers reported a bioconjugated nanoparticle-based biodetection for in situ pathogen quantification, which cost less than 20 min [120]. Tan’s success promises that quick and convenient pathogen detection is possible and can be achieved with these ingenious nanomaterials in the future. Here, we list diverse pathogens detected by fluorescent nanomaterials as shown in Table 2 [119, 121,122,123,124,125,126,127,128,129].
Nucleic Acid Detection
Apart from pathogen detection, fluorescent nanomaterials have also aroused more and more interest of scientists in the detection of DNA. Owing to the merits that a number of biomolecules can be attached to the surface of fluorescent nanomaterials, the signal intensity of fluorescent nanomaterials in DNA detection can be enhanced significantly. Tan and co-workers developed an DNA detection method to detect gene products using bioconjugated dye-doped fluorescent silica nanoparticle with high sensitivity and photostability [130]. Although the analysis of nucleic acid has been successfully achieved by real-time nanomaterial fluorescence systems, there are still many shortcomings such as complex procedures or expensive instruments. To address these disadvantages, Wang’s group introduced a highly sensitive and visualized detection of nucleic acid by the combination of strand exchange amplification (SEA) and lateral flow assay strip (LFA) [131]. The system, which is possible to be widely used in areas requiring limited resource, is mainly characterized by integrating SEA with LFA (Fig. 6). There is no denying that the extremely high fluorescent signal for bioanalysis plays an irreplaceable role in these applications.
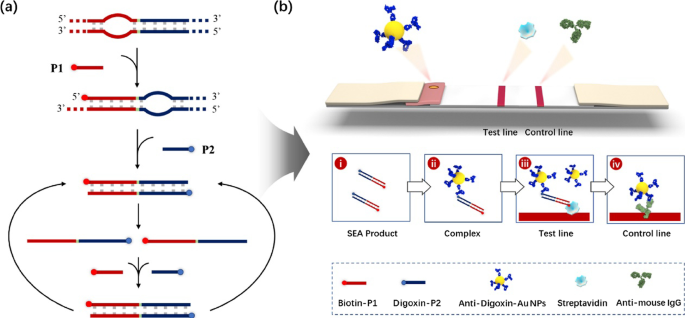
Schematic illustration of the SEA-LFA strip for the detection of nucleic acids [110]
Drug Detection
In the field of drug analysis, a facile and low-cost analytical method is always in demand for high-speed detection of specific pharmaceutical compounds. Real-time detection of drugs can be achieved with selective and sensitive fluorescent nanomaterials owing to their outstanding optical properties. In the past decade, the modification of nanomaterials has lowered their detection limit and improved their detection accuracy significantly. Recently, it is reported that ampicillin can be detected in serum sample based on aptamer, its complementary strand (CS) and gold nanoparticles (AuNPs) [132]. The limit of detection (LOD) of this method can be as low as 29.2 pM. However, there are still many limitations in the detection of drugs or target molecules in vivo. Due to low selectivity, conventional fluorescent nanomaterials inevitably generate false positive results and adverse effects in vivo. In addition, current tracking systems can hardly realize real-time tracking because of insufficient labels and excitation sources. Considering the above limitations, a new method using up/down conversion (UC/DC) PL nanomaterials has attracted increasing attention. Seo et al. reported a single-photon-driven UC/DC system which demonstrated outstanding performance in the detection of heavy metal ion (i.e. Hg 2+ ) in mussels [133]. LOD of the nanohybrids was ca. 1 nM. This system is appealing to researchers in the field of fluorescent nanomaterials for biomedical applications.
Drug Delivery
Until now, the technology of treating cancers with high efficiency and targeting function is not perfect enough. Under most circumstances, the anticancer drugs are distributed and released extensively in the body, which endangers the healthy cells and tissues irreversibly. Currently, a large variety of carriers for drug delivery have been designed. However, we can hardly supervise the distribution and result of the whole delivery process. Benefiting from the recent development of the surface modification technique, fluorescent materials capped with polymers like polyethylene glycol (PEG) can bond with drugs strongly and firmly. Then, the loaded drugs will be released in response to certain conditions such as pH, osmotic gradient and the surrounding environment. However, it should be confirmed whether the drugs are transported to the specific site or not. It’s also necessary for us to consider more details such as how much of the drugs is released in different positions. Aside from being drug carriers, fluorescent nanomaterials can also demonstrate the consequences of intracellular uptake due to their fluorescence property. QDs have been applied to monitor some important properties, such as delivery efficiency, release rate and distribution of drug molecules in vivo, which are beneficial for scientists in order to understand the specific targeting pathways of drug delivery within living cells. Duan and co-workers reported a facile pH-responsive fluorescent CDs drug delivery system [134]. Loaded with dox which is effective for gastric cancer, intracellular drug delivery and tracking could be simultaneously realized in patients (Fig. 7). The report highlighted the ability of fluorescent CDs to label and track the drug delivery process for at least 48 h, which showed a great potential in bioimaging, biolabeling and traceable drug delivery. Duan et al. designed a pH and receptor dual-responsive drug delivery system [135]. Hyaluronic acid was covalently attached to the surface of CDs, and doxorubicin was loaded by electrostatic self-assembly. In the tumor microenvironment (pH 5.6), the drug is released rapidly from the drug delivery system, while in the normal physiological environment (pH 7.4), the drug is hardly released. Endocytosis occurs when the drug delivery system reaches CD44 which is a receptor rich in tumor cells and can bind specifically to the hyaluronic acid. In addition, carbon nanotubes can be used for drug delivery by virtue of their high loading efficiency. Strong π-π interactions play a critical role in binding therapeutic agents with carbon nanotubes, which can be broke through changing external conditions, resulting in the release of drugs in specific position. Pennetta et al. functionalized single and multi-walled carbon nanotubes with a pyrrole derived compound to form a doxorubicin stacked drug delivery system. Biological studies showed that the synthesized nano-conveyors can effectively deliver the drug into cell lines and improve the therapeutic effects of doxorubicin [136].
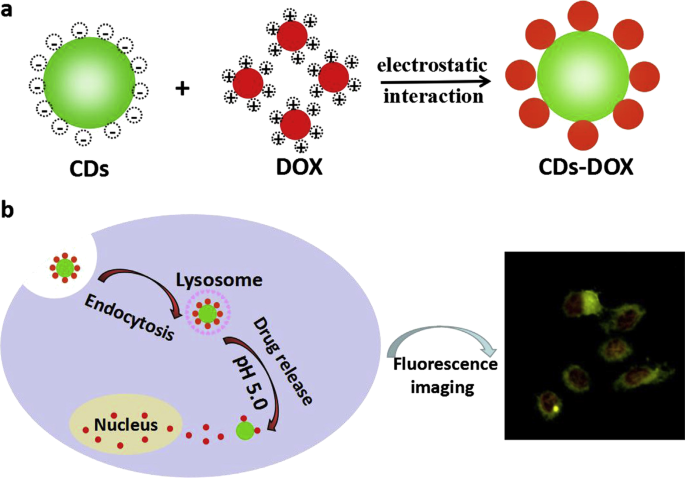
Schematic illustration of the preparation (a ) and cellular uptake (b ) of the CDs-DOX drug delivery system [113]
Photodynamic Therapy
Photodynamic therapy (PDT) is a novel therapy method for tumors which utilizes the interaction between light and photosensitizer. In PDT, reactive oxygen species (ROS) is produced from oxygen by photosensitizers in the condition of specific wavelengths of light (mostly in the area of near infrared light). The specific mechanism is presented in Fig. 8. ROS includes singlet oxygen, superoxide radicals, hydrogen peroxide, and hydroxyl radicals that possess strong cytotoxicity which cause significant destruction of tumor cells. However, there exist many defects such as limited penetration depth [137], hydrophobic properties [138], photobleaching [139], complicated procedure [140], and tumor hypoxia. PDT agents can hardly be dissolved and they will disperse extensively in vivo once they are taken, making it impossible to be targeted and selected. Fluorescent nanomaterial based photodynamic therapy developed fast in recent years [141]. Combined with the unique properties that QDs possess, such as high fluorescent efficiency and great spectral resolution, the effect of PDT can be enhanced. Barberi-Heyob, M and coworkers significantly enhanced the photodynamic efficiency with a concentration of 8 nM because of the light dose-dependent response [142]. In addition, photodynamic therapy can sometimes do harm to the skin and eyes of patients due to its photosensitive side-effect. To alleviate these adverse effects, a novel nanoparticle-based drug carrier for photodynamic therapy is reported which can provide stable aqueous dispersion of hydrophobic photosensitizers. Meanwhile, the key step of photogeneration of singlet oxygen was preserved, which is necessary for photodynamic action [143]. It is obvious that QDs combined photodynamic therapy will replace the conventional PDT someday.
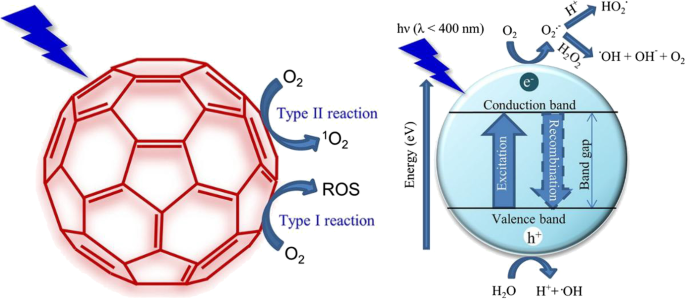
Schematic illustration of producing reactive oxygen species (ROS) for the photodynamic therapy (PDT) [119]
Challenges
Synthesis Challenges
Achieving Uniform Distribution
In the synthesis process, the diameter and size distributions of FNMs can be hardly distributed uniformly due to the agglomeration of small particles. This could be fatal to the optical properties of FNMs in biomedical application. For this reason, the applications of FNMs are still at the laboratory scale. It has been confirmed that the surface properties primarily determine the agglomeration state of the nanoparticles and their size. Therefore, surface modification is promising to achieve uniform distribution of FNMs by altering their surface properties [144]. To date, silanized QDs have been widely used because the polymerized silica coating increases the stability in buffers under physiological conditions [145]. Carbon dots synthesized by hydrothermal reaction using water-soluble base were reported to be difficult to control the size and distribution of grain boundary [146]. Khanam et al. reported a facile and novel synthetic method for the preparation of hydroxyl capped CDs using an organic base and a surfactant (Triton X-100) to modify the surface. A narrow particle size distribution at 7.2 nm was found in Raman and DLS studies, which is smaller than the majority of the particles falling within the range of below 10 nm in diameter [147].
Fluorescence Quantum Yield
Fluorescence quantum yield plays a crucial role for FNMs in their efficiency for on-demand light emission. Tunable and highly fluorescent CDs can be prepared with the surface functionalization approach. Nitrogen-doped FNMs are reported to have improved fluorescence quantum yield. With increasing nitrogen content, fluorescence quantum yield can be increased to as high as 56% at high synthesis temperature [148]. A facile strategy was also developed to tune the photoluminescent properties of CDs using a microwave irradiation, with citric acid and nitrogen-containing branched polyethyleneimine (b-PEI) as precursors. At intermediate levels of b-PEI, the CDs produced a high photoluminescence yield [149]. Lin et al. explored carbon dots with a high-fluorescence quantum yield rate synthesized from L-cysteine and citric acid by the microwave-assisted method. The obtained carbon dots exhibited a high-fluorescence quantum yield (up to 85%), which is due to the combination of amidogens and sulfydryl with carbon dots, and henceforth bringing the improved fluorescence property [150]. The above examples demonstrate that nitrogen or other electron-rich atoms like sulphur can obtain satisfying fluorescence quantum yield.
Aggregation-Caused Quenching
Fluorescent molecules can emit light with high efficiency in dilute solution. However, in concentrated solution or solid state, their fluorescence will be weakened or even disappear. This phenomenon is called Aggregation-Caused Quenching (ACQ) [151]. This problem has been puzzling scientists for almost 150 years, thus hindered the extensive application of fluorescent dyes.
In order to make effective use of fluorescent dyes, scientists have attempted many methods. Most of them focused on reducing the concentration of fluorescent dyes to prevent ACQ effect. Tang et al. discovered the phenomenon of Aggregation-Induced Emission (AIE) [152]. Based on rationally designed molecules, the fluorescence of organic molecules in solid state can be attained. Still, for more than one hundred thousand different fluorescent dyes in the world, the problem of ACQ has not been completely resolved. As long as they aggregate together, ACQ will make them lose their fluorescent properties.
It is almost impossible for high concentration or solid state FNMs to show reliable fluorescence due to fluorescence quenching. Although fluorescence quenching can be used as a sensitive signal to indicate substrate concentration in analytical chemistry, [153] in the most circumstances, however, fluorescence quenching is undesirable for FNMs because it always has considerable influence on bioimaging and biodetection. To overcome this long-standing problem, Benson et al. reported a universal solution with the discovery of a class of materials called small-molecule ionic isolation lattices (SMILES) [154]. SMILES are simple to make by mixing cationic dyes with anion-binding cyanostar macrocycles. We draw inspiration from their findings and believe that similar results can be obtained if we replace cationic dyes with cationic modified FNMs.
Application Challenges
Drawbacks of UV Light FNMs
Although FNMs realized the great-leap-forward from in vitro imaging to in vivo imaging, the emission fluorescence of most of FNMs is distributed in ultraviolet region or short wavelength visible region, which limits the optical imaging in living organisms. Moreover, use of UV light for monitoring living processes in cells and tissues has some potential drawbacks as long‐term irradiation of living cells may cause DNA damage and cell death. Therefore, the development of FNMs in near-infrared region is urgently needed in the future. Although NIR FNMs have deep tissue penetration, NIR detectors and filters are needed as the excitation and emission wavelengths are too close to each other, which restricts their range of application.
Interference in Biological Environment
Almost all biological tissues will produce significant autofluorescence under short wavelength, UV and visible light radiation [155]. Autofluorescence reduces the signal‐to‐background ratio and often interferes seriously with the visual effects. Some substances in the substrate of biological tissues also have great influence on the fluorescence, which reduces the selectivity of FNMs significantly. Until now, although the application of FNMs in mice showed acceptable outcomes, it is still difficult to achieve similar results in larger mammals. Much higher luminous efficiency under low power density excitation is required to avoid the background signal interference. Furthermore, temperature and pH conditions of the biological environments strongly affect the fluorescence of some substances as well. Therefore, satisfying fluorescence of FNMs at 37 °C and physiological pH should be guaranteed. It's worth noting that the pH in tumor is lower than normal tissues. Hence, fluorescence with high selectivity in acid environment will improve the efficiency of FNMs.
Biocompatibility
Biocompatibility refers to materials or systems that are nontoxic, safe and not causing physiological or immunological reactions. QDs with unique quantum confinement effect and electro-optical properties are attractive for biomedical applications. However, toxic effects of traditional semiconductor QDs made of heavy metal ions have serious safety concerns for their undesired environmental or health effects. In the purpose of circumventing this problem, core–shell structure modification of QDs by using biocompatible ligands or polymers is one way to effectively minimize toxic effects of traditional QDs. Furthermore, scientists are searching for heavy metal-free QDs formulations. Non-toxic or less toxic carbon dots and silica nanoparticles have shown their potential as the ideal FNMs for biomedical applications. Impurities brought in the process of syntheses may influence the biocompatibility of fluorescent nanomaterials. In order to reduce the influence of toxic impurities, green synthesis methods have been arousing the interest in biomedical fields. Chowdhury et al. utilized cacao extract which is a natural product as a reducing and stabilizing agent in the synthesis of gold nanoparticles [156]. Oxalic acid, as a constituent of cacao, can reduce Au 3+ in HAuCl4 to metallic gold and stabilize the resultant nanoparticle colloidal solution. In vitro studies suggested that the cacao derived gold nanoparticles are biocompatible and suitable for biomedical applications. For MOFs, appropriate metal ions and ligands must be selected to lower the toxicity. Wang et al. employed Fe, Ti and Zr as constituents of MOFs, which are harmless and even beneficial elements to the body [157]. In vitro studies indicate that the proposed material has good biocompatibility and safety in biomedical application. What’s more, it is necessary to consider whether the difference in composition, surface charge, or modified group will have different biological effects. Taking these factors into account, we can improve the biocompatibility of FNMs with rational design.
Conclusions
Benefiting from the unique properties of fluorescent nanomaterials, some limitations and barriers of conventional materials and methods in biomedical applications can be broken through. In this review, we comprehensively present the synthesis methods and applications of fluorescent nanomaterials. The advanced synthesis methods can offer us the fluorescent nanomaterials with ideal morphology, size ranges and structures. Meanwhile, the more convenient syntheses can lower the manufacturing cost of fluorescent nanomaterials, which is critical to their widespread applications in biomedical fields. Based on the improved synthesis techniques, the performance of fluorescent nanomaterials is bound to leap in their applications. With the development of fluorescent nanomaterials, bioimaging, biodection, drug delivery and photodynamic therapy will be more widely applied in the diagnosis and treatment of diseases. Finally, challenges in synthesis and biomedical applications point out exiting questions and developing direction. We hope that this review can bring some new insights to the development of fluorescent nanomaterials.
Доступность данных и материалов
All data and materials are available without restrictions.
Сокращения
- FNMs:
-
Fluorescent nanomaterials
- PL:
-
Photoluminescence
- CDs:
-
Carbon dots
- QDs:
-
Quantum dots
- b-PEI:
-
Branched polyethyleneimine
- SWCNTs:
-
Single-walled carbon nanotubes
- CQDs:
-
Carbon quantum dots
- GQDs:
-
Graphene quantum dots
- NCQDs:
-
Nitrogen-doped carbon quantum dots
- NSCDs:
-
Nitrogen and sulfur doped carbon dots
- QY:
-
Quantum yield
- MCDs:
-
Multi-doped carbon dots
- MWCHTs:
-
Multi-walled carbon nanotubes
- GO:
-
Graphene oxide
- rGO:
-
Reduced graphene oxide
- CVD:
-
Химическое осаждение из паровой фазы
- AuNPs:
-
Gold nanoparticles
- Ag NCs:
-
Ag nanoclusters
- Cu NCs:
-
Cu nanoclusters
- NPs:
-
Nanoparticles
- TEOS:
-
Tetraethylorthosilicate
- MR:
-
Magnetic resonance
- COFs:
-
Covalent-organic frameworks
- TNP:
-
2,4,6-Trinitrophenol
- MOFs:
-
Metal organic frameworks
- ACQ:
-
Aggregation-caused quenching
- NIR:
-
Near infrared
- SEA:
-
Strand exchange amplification
- LFA:
-
Lateral flow assay strip
- CS:
-
Complementary strand
- LOD:
-
Limit of detection
- UC:
-
Upconversion
- DC:
-
Downconversion
- PDT:
-
Photodynamic therapy
- ROS:
-
Reactive oxygen species
- AIE:
-
Aggregation-induced emission
- SMILES:
-
Small-molecule ionic isolation lattices
Наноматериалы
- Датчики и процессоры объединяются для промышленного применения
- Что будет дальше с 3D-печатью - биомедицинские приложения
- Пять основных проблем и проблем для 5G
- Наночастицы для терапии рака:текущий прогресс и проблемы
- Графен и полимерные композиты для суперконденсаторов:обзор
- Последние достижения и приложения в технологии Интернета вещей
- EconCore лицензирует Fynotej для автомобильных приложений и продвигается в области производства высокопроизводите…
- Приводы для промышленного охлаждения и промышленного охлаждения
- Токарно-карусельные станки для расточной и токарной обработки
- Понимание преимуществ и проблем гибридного производства